Nanopartículas de oro multifuncionales para aplicaciones terapéuticas y diagnósticas mejoradas:una revisión
Resumen
Las propiedades médicas de los metales se han explorado durante siglos en la medicina tradicional para el tratamiento de infecciones y enfermedades y todavía se practican hasta la fecha. Los medicamentos a base de platino son la primera clase de medicamentos a base de metales que se utilizan clínicamente como agentes contra el cáncer tras la aprobación del cisplatino por la Administración de Alimentos y Medicamentos de los Estados Unidos (FDA) hace más de 40 años. Desde entonces, se han aprobado más metales con beneficios para la salud para ensayos clínicos. Curiosamente, cuando estos metales se reducen a nanopartículas metálicas, mostraron propiedades únicas y novedosas que eran superiores a sus contrapartes a granel. Las nanopartículas de oro (AuNP) se encuentran entre las nanopartículas metálicas aprobadas por la FDA y han demostrado ser muy prometedoras en una variedad de funciones en la medicina. Se utilizaron como agentes de administración de fármacos, fototérmicos (PT), de contraste, terapéuticos, radiosensibilizantes y de transfección de genes. Sus aplicaciones biomédicas se revisan aquí, cubriendo su uso potencial en el diagnóstico y la terapia de enfermedades. También se analizan algunos de los sistemas basados en AuNP que están aprobados para ensayos clínicos, así como las amenazas potenciales para la salud de los AuNP y algunas estrategias que pueden usarse para mejorar su biocompatibilidad. Los estudios revisados ofrecen una prueba de principio de que los sistemas basados en AuNP podrían potencialmente usarse solos o en combinación con los sistemas convencionales para mejorar su eficacia.
Introducción
La medicina es uno de los muchos campos que se han beneficiado de la nanotecnología. La nanotecnología surgió con muchas oportunidades para mejorar y desarrollar nuevos agentes terapéuticos y de diagnóstico mediante el uso de nanomateriales [1, 2]. Los AuNP, en particular, exhiben propiedades fisicoquímicas únicas y una buena estabilidad química. Son fáciles de funcionalizar con casi todos los tipos de moléculas donantes de electrones, a través de diversas químicas o en función de su fuerte afinidad por las moléculas tioladas [3, 4]. Debido a su pequeño tamaño, los AuNP tienen una superficie más grande y una alta capacidad de carga de fármacos. Pueden incorporarse múltiples restos en las AuNP para aplicaciones biomédicas; estos incluyen moléculas dirigidas para aumentar la especificidad, agentes de contraste para bioimágenes y para monitorear la respuesta de la enfermedad a los fármacos en tiempo real, y agentes terapéuticos para el tratamiento de enfermedades [5, 6]. Curiosamente, incluso sin biomoléculas añadidas, las AuNP son capaces de apuntar, obtener imágenes y tratar enfermedades. Basándose en sus propiedades dependientes del tamaño, se pueden crear nuevos sistemas basados en AuNP para su uso en diversas aplicaciones biomédicas [7].
Los AuNP están hechos de un precursor de metal que es termoestable y, por lo tanto, son muy estables y no biodegradables. El oro a granel se utiliza en medicina y se ha demostrado que es bioinerte y no tóxico [8, 9]; por lo tanto, el núcleo de oro en los AuNP esencialmente mostrará propiedades similares [3, 10]. Las AuNP y sus aplicaciones se han estudiado ampliamente durante más de cinco décadas y han demostrado ser muy prometedoras como agentes teranósticos en estudios preclínicos [5, 11,12,13] y clínicos [14,15,16,17,18]. Existen muchas más oportunidades para nuevos sistemas basados en AuNP, como se analiza en esta revisión. Las AuNP ya se han explorado en ensayos clínicos como portadores de fármacos para el tratamiento de cánceres en etapa tardía [16, 17] y como agentes de TP en el tratamiento del cáncer de próstata [19] y el acné [18]. Sin socavar las cuestiones sanitarias y reglamentarias que rodean el uso de AuNP [20], el futuro de estos sistemas en biomedicina está al alcance de la mano. Son posibles los sistemas multifuncionales basados en AuNP que son capaces de combatir la resistencia a los fármacos con una eficacia localizada y mejorada [11, 21, 22]. La revisión destaca las propiedades biológicas de las AuNP en estudios preclínicos y clínicos, al reflexionar sobre sus bioaplicaciones como agentes diagnósticos y terapéuticos. También se describen las posibles amenazas para la salud y las estrategias que se utilizaron para superar sus limitaciones. Finalmente, se destacan las perspectivas de futuro de las AuNP en medicina.
Nanopartículas de oro
La popularidad de los AuNP en aplicaciones médicas ha ganado mucho impulso debido a sus propiedades químicas y físicas únicas. Las AuNP son partículas coloidales sólidas que varían en tamaño de 1 a 100 nm [23]. Las aplicaciones de las AuNP en biología se basan en sus propiedades fisicoquímicas, sin limitarse a su tamaño, resonancia de plasmón superficial (SPR), forma y química de superficie [3, 10]. Estos parámetros influyen en su actividad y los convierten en candidatos perfectos para su uso en el diagnóstico y tratamiento de enfermedades, ya sea como agentes de administración, sensibilizantes, contrastes o terapéuticos. Su pequeño tamaño se asocia con un área de superficie más grande, lo que permite la modificación de la superficie y la unión de múltiples cargas útiles, como agentes terapéuticos, de imagen y de orientación [4, 24, 25, 26]. Su pequeño tamaño también hace posible que las NP y su carga atraviesen barreras biológicas que de otra manera serían difíciles de alcanzar y penetrar [11].
Las AuNP se reconocen cada vez más como agentes diagnósticos, terapéuticos y teranósticos (un agente que puede usarse simultáneamente para diagnosticar y tratar una enfermedad), que tienen potencial para abordar los efectos fuera del objetivo asociados con las terapias convencionales. Sin embargo, los AuNP poseen propiedades y funciones diferentes en comparación con sus homólogos a granel biocompatibles, que podrían ser peligrosos para la salud humana [27, 28, 29]. El uso clínico de compuestos de oro a granel para el tratamiento de enfermedades es una práctica antigua y está certificado como seguro [8]. En los últimos años, la investigación ha demostrado que los AuNP tienen propiedades médicas similares o mejoradas [29]. Debido a sus propiedades ópticas, químicas y físicas únicas, los AuNP a menudo presentan propiedades novedosas en comparación con el oro a granel [30, 31] y pueden servir como agentes de diagnóstico y terapéuticos [5].
Síntesis de AuNP
Los AuNP se pueden producir de varias formas siguiendo el enfoque de arriba hacia abajo o de abajo hacia arriba. El enfoque de arriba hacia abajo utiliza métodos físicos y químicos para producir los tamaños deseados a partir del material a granel, mientras que el enfoque de abajo hacia arriba implica métodos químicos para ensamblar los componentes básicos en la formación de sistemas nanométricos [32, 33]. Los métodos físicos (como molienda, fotoquímica, radiación y litografía) utilizan una gran cantidad de energía y presión para reducir los materiales a granel a 10 –9 mil millonésima parte de un metro de tamaño [10, 32, 34]. Los procesos de nucleación se controlan fácilmente cuando se utilizan los métodos físicos, no se requieren agentes reductores y con algunos de estos métodos la síntesis ocurre simultáneamente con la esterilización de los NP. Sin embargo, las tecnologías físicas a menudo son costosas, no están fácilmente disponibles y requieren equipo especializado. Además, es posible que los agentes de protección y estabilización no sobrevivan a los procesos de alta energía involucrados en estos procesos [34].
El enfoque ascendente se prefiere principalmente en la síntesis de AuNP, ya que es rápido, fácil y no requiere el uso de equipos sofisticados [33,34,35]. Se basa en el método químico desarrollado por Turkevich en 1951 (Fig. 1A), que utiliza citrato para la reducción y estabilización de un precursor de oro, lo que da como resultado la producción de AuNP esféricos de 15 nm [3, 10, 23, 33, 36 , 37]. El método se modificó aún más variando la proporción de citrato al contenido de precursor de oro y dio como resultado un rango de diámetro de tamaño de 15-150 nm AuNPs (Fig. 1B) [10, 24]. También se introdujeron varios agentes reductores, como borohidruro de sodio, bromuro de cetiltrimetilamonio (CTAB) y ácido ascórbico. Algunos de los agentes reductores químicos son, lamentablemente, tóxicos [33, 34, 36] y normalmente se pasivan añadiendo agentes estabilizantes en su superficie, como polietilenglicol (PEG), goma arábiga, polisacáridos y péptidos bioactivos [37, 38].
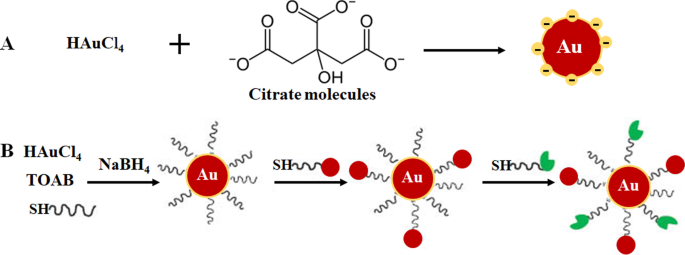
Formulación de AuNP mediante sistema monofásico por reducción de citrato ( A ) y reducción del sistema en dos fases seguida de estabilización y funcionalización mediante la reacción de intercambio de ligandos, método de Brust-Schiffrin ( B ). Reproducido con autorización [36]. Copyright 2013, De Gruyter. TOAB bromuro de tetrabutilamonio, SH moléculas tioladas
Se han explorado enfoques más ecológicos, como el proceso de plasma en líquido inducido por microondas (MWPLP) y la nanotecnología verde, en las AuNP de síntesis para evitar el uso de agentes reductores químicos tóxicos. El MWPLP utiliza microondas para generar nucleación de NP metálicas y no requiere ningún agente reductor, y la energía requerida para la síntesis es muy baja [34]. La nanotecnología verde, por otro lado, utiliza compuestos naturales procedentes de plantas y microorganismos como fuente de agentes reductores en la síntesis de AuNP biogénicos [12, 33, 39,40,41]. La nanotecnología verde se considera ecológica y respetuosa con el medio ambiente y, por lo tanto, más adecuada para aplicaciones biomédicas. La síntesis mediada por plantas es más económica que el uso de microorganismos. Además, la síntesis se puede realizar en un solo paso y las NP son más fáciles de purificar. Además, las plantas son renovables; Varias partes de las plantas, como hojas, tallos, cortezas, raíces, flores y frutos, pueden cosecharse sin matar la planta y usarse para síntesis. Los extractos preparados a partir del material vegetal contienen fitoquímicos, proteínas y enzimas que pueden funcionar como agentes reductores, estabilizantes y protectores [10, 12, 24, 34, 35, 40, 42]. La epigalocatequina de los tés verdes [42] y la mangiferina (MGF) de los mangos [12, 43] se encuentran entre los compuestos derivados de plantas que se han utilizado ampliamente para sintetizar AuNP [34]. Más información sobre estos métodos se revisa extensamente en las siguientes referencias [10, 24, 34, 35].
Aplicación biológica de AuNPs
El papel y la importancia de las AuNP en la ciencia médica, sin duda, se están volviendo más visibles, lo que está respaldado por el número cada vez mayor de estudios que demuestran su aplicación multifacética en una amplia gama de campos biomédicos. La biocompatibilidad de los AuNP se atribuye a la larga historia del oro en el tratamiento de enfermedades humanas, que se remonta al 2500-2600 a. C. Los chinos e indios usaban oro para el tratamiento de la impotencia masculina, la epilepsia, la sífilis, las enfermedades reumáticas y la tuberculosis. China descubrió el efecto de longevidad del oro coloidal rojo, que todavía se practica en la India como parte de la medicina ayurvédica para el rejuvenecimiento y la revitalización. El cinabrio-oro (también conocido como Makaradhwaja) se usa para mejorar la fertilidad en la India. En los países occidentales, el oro se ha utilizado para tratar los trastornos nerviosos y la epilepsia. No se informó toxicidad por su uso tanto en estudios in vitro como in vivo [8, 44, 45]. Desde entonces, los compuestos de oro orales e inyectables continuaron utilizándose como tratamientos para la artritis [9, 46] y también se ha demostrado que tienen efectos anticancerígenos [8]. También se informaron efectos similares y, en algunos casos, mejorados para las AuNP, que están emergiendo como agentes prometedores para el diagnóstico de enfermedades [47, 48, 49] y la terapia [3, 29, 50, 51].
Los AuNP tienen un área de superficie más grande que se puede explotar para aplicaciones biomédicas, al unir varias biomoléculas para adaptarse a una función deseada. Estos pueden incluir grupos de orientación para ayudar a reconocer biomarcadores específicos de la enfermedad, agentes de contraste para la obtención de imágenes biológicas y agentes terapéuticos para el tratamiento de enfermedades [24, 25]. La ventaja de utilizar AuNP sobre otros nanomateriales es que se pueden funcionalizar fácilmente utilizando diversas químicas, como se demuestra en la Fig. 2 [4, 26]. Los AuNP tienen una alta afinidad por las moléculas tioladas, y la unión de tiol-oro es el método más utilizado para adsorber moléculas en la superficie del NP [4]. También se utilizan químicas basadas en afinidad tales como unión de biotina-estreptavidina y acoplamiento de carbodiimida. Las AuNP se utilizan en tres áreas principales de la biomedicina:administración de productos farmacéuticos, con fines diagnósticos y terapéuticos [24, 35], y han demostrado un enorme potencial en estas áreas, como se analiza a continuación.
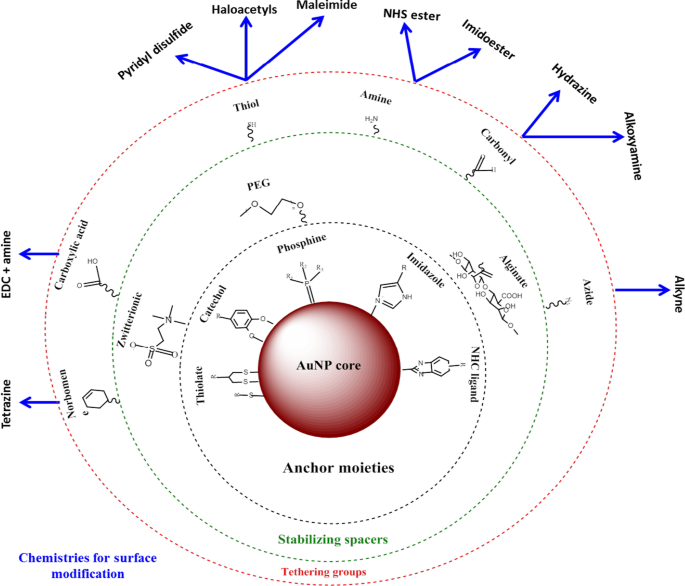
Síntesis y funcionalización de AuNPs. Las biomoléculas con grupos funcionales se adsorben primero en la superficie del NP a través de la afinidad oro-tiol. Luego, se pueden usar otros grupos funcionales, como el grupo amina, para unir moléculas con grupos carboxilo para unir restos de fármacos o de dirección. Adaptado de [32]
AuNP como agentes de administración de medicamentos
La aplicación más común de las AuNP es como vehículos de administración de fármacos [11, 18, 52], vacunas [53] y terapia génica [24, 32]. Las AuNP poseen propiedades que pueden resolver la mayoría de los problemas asociados con las terapias convencionales, como la resistencia a los fármacos, la baja distribución de los fármacos, la biodegradación y la eliminación temprana del fármaco [11]. Las AuNP pueden reducir significativamente la dosis de los medicamentos, la frecuencia del tratamiento y son capaces de transportar medicamentos hidrófobos e insolubles. Se considera que son bioinertes y pueden enmascarar su cargamento del ataque de las células inmunitarias, proteger a los fármacos de la degradación proteolítica a medida que viajan a través del sistema circulatorio y, por lo tanto, aumentar el tiempo de circulación del fármaco. Estos factores pueden aumentar fácilmente la eficacia de los fármacos al concentrarlos y retenerlos en los tejidos enfermos con poco o ningún efecto sobre los tejidos normales [25].
El uso de AuNP en el tratamiento del cáncer se ha estudiado ampliamente [17, 37, 54] y, a lo largo de los años, se ha extendido a otras enfermedades como la obesidad [50, 55, 56] y el acné [18]. Los sistemas basados en nano son más pequeños que la mayoría de los componentes celulares y pueden atravesar pasivamente las barreras celulares aprovechando el efecto de mayor permeabilidad y retención (EPR) en la vasculatura de los tejidos enfermos [25]. La EPR en un estado patológico se caracteriza por una angiogénesis excesiva y un aumento de la secreción de mediadores de la permeabilidad, que pueden mejorar la captación de AuNP por los tejidos enfermos. Estas características solo se asocian con estados patológicos y no con tejidos normales, que brindan una oportunidad para el direccionamiento selectivo de los conjugados de AuNP [25]. Los AuNP son atractivos como portadores de fármacos, ya que pueden transportar múltiples moléculas simultáneamente, diversificando aún más sus propiedades. Este es un rasgo deseable en medicina en el que se basan la mayoría de las bioaplicaciones de AuNP, ya que las AuNP se pueden adaptar para una función biomédica específica. Esto puede ayudar a controlar la forma en que interactúan con los orgánulos celulares y, por lo tanto, es prometedor para el desarrollo futuro de modalidades de diagnóstico y tratamiento eficaces para diversas enfermedades [4].
Sistemas de diagnóstico basados en AuNP
La aparición de la nanotecnología ha elevado la posición en el desarrollo de sistemas de detección que sean rápidos, robustos, sensibles y altamente competitivos en comparación con las pruebas de diagnóstico convencionales [48]. Los nanomateriales suelen estar integrados en las plataformas de biosensores existentes para la detección de marcadores de gases, ADN y proteínas implicados en el desarrollo de enfermedades [47]. Entre los diversos nanomateriales (que incluyen NP metálicos, poliméricos, magnéticos y semiconductores) utilizados en el diagnóstico, los AuNP se han utilizado ampliamente en biosensores, sensores electroquímicos y ensayos cromogénicos para detectar o detectar la presencia de biomarcadores de enfermedades [49]. Su SPR localizado (LSPR), transferencia de energía de resonancia de fluorescencia (FRET), dispersión Raman mejorada en la superficie, conductividad, actividad redox y efecto de carga cuantificado los convierten en una herramienta ideal para la formación de imágenes y la detección de moléculas objetivo [10, 24]. Sus propiedades electrónicas y ópticas y su capacidad para dispersar la luz visible y en el infrarrojo cercano (NIR) son compatibles y medibles con diversas tecnologías, como las técnicas microscópicas (dispersión de luz electrónica, confocal y de campo oscuro) [57], tomografía computarizada (TC) , PT técnica de imagen heterodina, espectroscopia UV-Vis y Raman [24, 35].
El desarrollo de sistemas de diagnóstico basados en AuNP implica la modificación de la superficie de AuNP, por ejemplo, mediante la unión de biomoléculas que reconocen biomarcadores de enfermedades [3, 24, 58]. Los ensayos de flujo lateral (LFA) son probablemente el ejemplo más conocido de herramientas de diagnóstico basadas en nanotecnología. Los LFA suelen utilizar AuNP de aproximadamente 30-40 nm porque las partículas más pequeñas tienen secciones transversales de extinción muy pequeñas, mientras que las partículas más grandes suelen ser inestables para su uso en estos ensayos [59]. Además, se incluyen otras moléculas / enzimas que pueden desencadenar cambios en SPR, conductividad y redox de AuNP. Estos indicadores dan una señal detectable después de la unión de analitos a los conjugados AuNP [24], la falta o presencia de señal reflejará la ausencia o presencia de la molécula diana o la enfermedad. La señal generada por AuNPs es químicamente estable, duradera y consistente cuando se usa en diferentes formatos de prueba:probeta, tira, in vitro e in vivo [24]. Por lo tanto, su aplicación ha aumentado notablemente la velocidad y el éxito de los ensayos de diagnóstico.
Ensayos colorimétricos basados en AuNP
En los ensayos colorimétricos, los AuNP producen una señal visual (generalmente un cambio de color) que se puede detectar a simple vista sin el uso de instrumentos avanzados. Generalmente, una solución coloidal de AuNPs tiene un color rojo rubí a uva que depende en gran medida de la distancia entre partículas [60, 61]. La unión de un analito a los AuNP modificados con elementos de bioreconocimiento molecular (p. Ej., Anticuerpos, péptidos, aptámeros, enzimas, etc.) induce un cambio distinto en el LSPR, lo que da como resultado el cambio de color del rojo rubí al azul [60 , 62, 63]. La intensidad del color es directamente proporcional a la concentración de un analito y se utiliza para confirmar la presencia y el estado de la enfermedad. Los diagnósticos colorimétricos basados en AuNP se han utilizado con éxito en la detección del virus de la influenza A [64], el virus del Zika [65], el bacteriófago T7 [66], Mycobacterium tuberculosis [67], y recientemente, para la detección del síndrome respiratorio agudo severo-coronavirus-2 (SARS-CoV-2) [60, 68].
Se demostró un ejemplo de un ensayo colorimétrico basado en AuNP para la detección de SARS-CoV-2 [60], un virus que causa una enfermedad de coronavirus 2019 altamente infecciosa (COVID-19) [60, 68]. Con este ensayo, la presencia del virus se informó mediante un simple cambio de color; no se requirió instrumentación para hacer el diagnóstico. Las pruebas de diagnóstico clínico actuales de este virus utilizan el ensayo de reacción en cadena de la polimerasa en tiempo real con transcriptasa inversa (RT-PCR), que toma de 4 a 6 h, mientras que los sistemas rápidos de punto de atención (PoC) detectan anticuerpos que podrían tomar varios días para aparecer en la sangre. En comparación, el ensayo colorimétrico basado en AuNP fue más robusto y rápido, como se demuestra en la Fig. 3. La incubación de AuNP marcados con oligonucleótidos antisentido (ASO) en presencia de muestras de ARN del SARS-CoV-2 resultó en la formación de un precipitado azul dentro ~ 10 min. En una prueba de SARS-CoV-2 positiva, la unión de los ASO al gen N en la fosfoproteína de la nucleocápside del virus indujo un color azul que se detectó visualmente. La prueba fue muy sensible y tenía un límite de detección de 0,18 ng / μL para el ARN del SARS-CoV-2 [60].
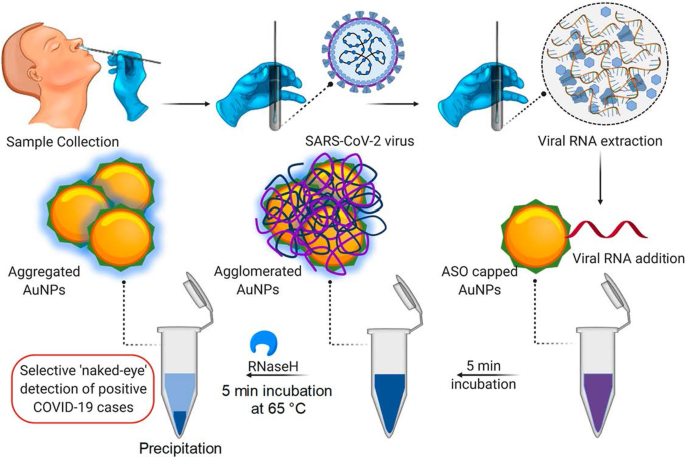
Sistema de diagnóstico colorimétrico basado en AuNP. Detección selectiva a simple vista del ARN del SARS-CoV-2 por los AuNP cubiertos con ASO. Reproducido con autorización [60]. Copyright 2020, ACS Nano
Los LFA basados en AuNP siguen el mismo principio que el que se muestra en la Fig. 3; sin embargo, en lugar de un cambio de color en una solución, se forma una línea visible en una tira reactiva cuando hay un analito presente. En presencia de un analito, los AuNP se capturaron en la línea de prueba y formaron una línea roja distinta, que se visualizó a simple vista. La intensidad de la línea está determinada por el número de AuNP adsorbidos [69]. En la figura 4 se muestra un ejemplo de un LFA basado en AuNP simple y rápido para la detección de Pneumocystis jirovecii ( P. jirovecii ) Anticuerpos IgM en sueros humanos. Los AuNP de 40 nm se conjugaron con los antígenos sintéticos recombinantes (RSA) de P. jirovecii , ya sea la glicoproteína de superficie principal o la serina proteasa de tipo kexina, que se utilizaron como indicador de la presencia o ausencia de P. jirovecii . En una prueba positiva, el P. jirovecii La IgM fue capturada por el conjugado AuNP-RSA en la almohadilla del conjugado. El complejo AuNP-RSA / IgM luego fluye a la membrana analítica donde se une al anti-IgM humano (línea de prueba) y el exceso se mueve a los anticuerpos anti-RSA (línea de control), lo que da como resultado dos líneas rojas. La prueba negativa solo tendrá un color rojo en la línea de control [70]. Un estudio independiente utilizó el LFA basado en AuNP para detectar selectivamente la IgM del SARS-CoV-2, como lo confirma la aparición de las líneas rojas en las líneas de prueba y de control [68]. El color se detectó visualmente a simple vista en 15 minutos en los dos sistemas, y solo se necesitaron muestras de suero de 10 a 20 μL por prueba [68, 70].
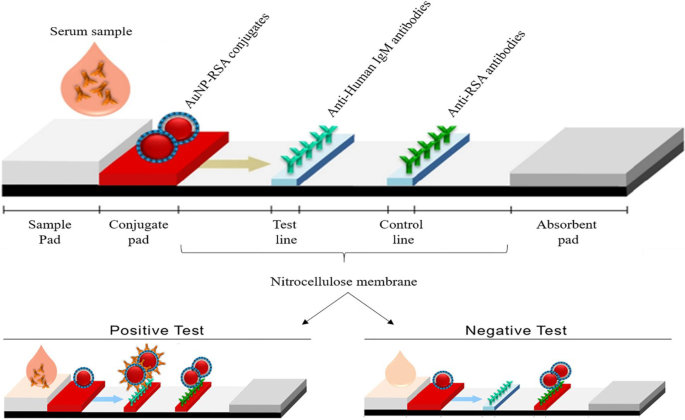
LFA basados en AuNP para la detección de IgM P. jirovecii anticuerpos. La presencia (prueba positiva) o ausencia (control negativo) de P. jirovecii los anticuerpos podrían diferenciarse por el color rojizo de AuNP tanto en la línea de prueba como en la de control, o solo en la línea de control, respectivamente. Reproducido con autorización [70]. Copyright 2019, Fronteras en microbiología
Uno de los primeros ejemplos del uso de AuNP como sonda de señalización en un LFA fue para la detección de células de Ramos; el aptámero TE02 se utilizó como sonda de captura y el aptámero TD05 como sonda de detección. El biosensor aptámero-AuNP puede detectar visualmente un mínimo de 4000 células Ramos sin instrumentación y 800 células Ramos con un lector de tiras portátil en 15 minutos. Usando este biosensor de detección sándwich, el ensayo detectó con éxito células de Ramos enriquecidas en sangre humana [71] y se utilizó como prueba de concepto para desarrollar sistemas rápidos, sensibles y de bajo costo para la detección cualitativa y cuantitativa de células cancerosas circulantes. Desde entonces, se han diseñado varios LFA basados en AuNP para el diagnóstico de numerosas enfermedades infecciosas, incluidas las enfermedades causadas por neumonía por Pneumocystis [70], virus del Ébola [72], VIH, virus de la hepatitis C y Mycobacterium tuberculosis [73] y más recientemente el virus SARS-CoV-2 [68].
Sistemas de imágenes basados en AuNP
Las AuNP se han investigado intensamente para aplicaciones en bioimágenes debido a su capacidad para absorber y dispersar luz que coincida con sus longitudes de onda de resonancia, hasta 10 5 veces más que los fluoróforos convencionales [74]. Los AuNP tienen un número atómico y una densidad de electrones más altos (79 y 19,32 g / cm 3 ) en comparación con los agentes convencionales a base de yodo (53 y 4,9 g / cm 3 ), demostrando así ser mejores agentes de contraste [24]. Las AuNP se acumulan en las células o tejidos enfermos e inducen una fuerte atenuación de los rayos X, lo que hace que el sitio objetivo sea muy distinto y fácilmente detectable. Los AuNP se unen a restos químicos y agentes de bioreconocimiento molecular que pueden apuntar selectivamente a antígenos específicos para inducir un contraste distinto y específico para el objetivo para la obtención de imágenes por TC [75].
El sistema de obtención de imágenes de CT molecular dirigida in vitro se logró mediante el uso de AuNP funcionalizados con un aptámero de ARN que se une al antígeno de membrana específico de la próstata (PSMA). El conjugado de aptámero AuNP-PSMA mostró más de cuatro veces la intensidad de CT para las células de próstata que expresan PSMA (LNCaP) en comparación con las células de próstata PC-3, que carecen del receptor diana [76]. De manera similar, el conjugado AuNP-ácido diatrizoico-aptámero AS1411 se localizó en células CL1-5 (adenocarcinoma de pulmón humano) y ratones portadores de tumores CL1-5. El aptámero AS1411 se dirige al receptor de nucleolina (NCL) que es expresado por las células CL1-5 en la superficie celular, mientras que el ácido diatrizoico es un agente de contraste a base de yodo. El conjugado AuNP-ácido diatrizoico-aptámero AS1411 tenía una curva de atenuación lineal con una pendiente de 0,027 mM de unidades Au Hounsfield (HU −1 ) que indica la acumulación de AuNP en el sitio del tumor [77]. Los AuNP exhibieron un tiempo de retención vascular más largo, lo que prolongó su tiempo de circulación en la sangre [77, 78, 79] y mejoró la señal de TC del ácido diatrizoico [77].
La Figura 5 muestra una imagen vascular por TC in vivo de arterias coronarias utilizando AuNP que se conjugaron con la proteína de adhesión 35 (CNA35) de unión a colágeno para dirigirse al colágeno I en el infarto de miocardio en roedores. La señal de AuNP todavía se detectó en la sangre 6 h después de la administración intravenosa (i.v), que fue significativamente más alta que la vida media (5-10 min) de los agentes a base de yodo [79]. Estos efectos se replicaron mediante el uso de AuNP cubiertas con manano sintetizadas en verde, que mostraron captación mediada por receptor y no toxicidad en células que expresan manosa (DC 2.4 y RAW 264.7). Las AuNP cubiertas con manano se dirigieron selectivamente a los ganglios linfáticos poplíteos in vivo después de la inyección en la pata trasera de los ratones [38]. Las imágenes de TC basadas en AuNP pueden proporcionar información significativa para el diagnóstico de diversas enfermedades que no se limitan a las arterias coronarias y los cánceres [76, 77, 79,80,81]. El uso de AuNP como agentes de contraste ha mostrado potencial en otros sistemas de imágenes como la imagen fotoacústica, la imagen nuclear, la ecografía y la resonancia magnética. Estos sistemas se revisan extensamente en otros lugares [82, 83].
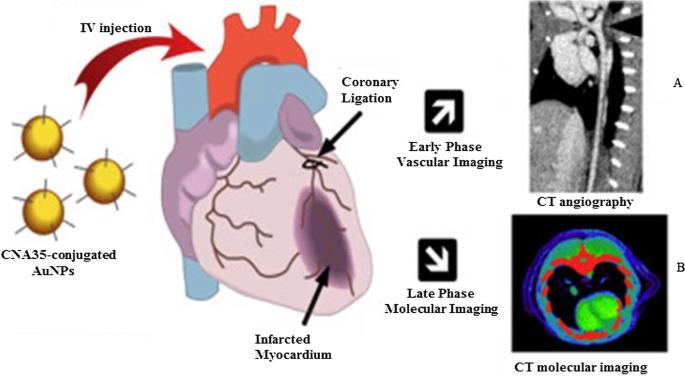
Imágenes de TC in vivo utilizando AuNP como agentes de contraste de TC. AuNP con tapa de manano y sus imágenes por TC del ganglio linfático ( A ) e imágenes de TC de AuNP conjugadas con CNA35 de la carga de cicatrices del miocardio ( B ). Reproducido con autorización [79]. Copyright 2018, Elsevier
AuNP en sistemas de detección basados en fluorescentes
Los AuNP se utilizan en sistemas de detección basados en fluorescencia como agentes fluorescentes o extintores de fluorescencia. En tamaños ≤ 5 nm, los AuNP muestran las propiedades de los puntos cuánticos (QD) y se pueden usar en su lugar. El Au 55 (PPh 3 ) 12 Cl 6 Los nanoclusters introducidos en 1981 son probablemente los más estudiados debido a su comportamiento de tamaño cuántico [7]. Desde entonces, varios AuNP de tamaño cuántico (AuNPsQ) como Au 25 (SR) 18 , Au 38 (SR) 24 y Au 144 (SR) 60 [84] se han estudiado principalmente en detección electroquímica, ya que son excelentes conductores electrónicos y mediadores redox [85].
Se utilizaron electrodos de película AuNPsQ en la fabricación de un inmunosensor electroquímico ultrasensible para la detección de antígeno prostático específico (PSA). El inmunosensor tenía una sensibilidad de 31,5 μA mL / ng y un límite de detección de 0,5 pg / mL para PSA en 10 μL de suero humano sin diluir. El inmunoensayo funcionó ocho veces mejor que un inmunosensor de bosque de nanotubos de carbono previamente informado que contenía múltiples fracciones, a una concentración de biomarcador que era más baja que los niveles asociados con la presencia de cáncer. Como tal, puede usarse para medir el biomarcador de prueba tanto en estados normales como enfermos. El rendimiento del inmunosensor fue comparable al del método ELISA de referencia [86]. AuNPsQ también se incorporó en CaCO 3 de estructura porosa esferas para formar un CaCO 3 fluorescente / Híbrido AuNPsQ para la detección de enolasa específica de neuronas, un biomarcador de diagnóstico y pronóstico para el traumatismo craneoencefálico y el cáncer de pulmón. El sensor tenía un límite de detección de 2.0 pg mL −1 [87]. Hasta ahora, se han informado varios sistemas de detección fluorescente basados en AuNP para la detección de analitos asociados con la hepatitis B [73, 88], la influenza A [89], el cáncer [90] y las lesiones cardíacas [91].
Los AuNP también son excelentes extintores basados en FRET [92]. Sus propiedades ópticas únicas (intensidad de señal estable y resistencia al fotoblanqueo), tamaño y capacidad de modificación las han convertido en sondas atractivas en plataformas de detección de fluorescencia [93, 94]. Los AuNP más grandes (≥ 10–100 nm) tienen rendimientos cuánticos bajos que no son adecuados para la detección fluorescente directa; sin embargo, su capacidad para apagar los tintes fluorescentes en un estado de energía de excitación relativamente alta los ha convertido en extintores de fotoluminiscencia eficaces [94]. En principio, las nanosensas de fluorescencia están compuestas por un fluoróforo donante (tinte o QD) y un AuNP aceptor, y cuando se acercan, la fluorescencia del fluoróforo seleccionado es apagada por los AuNP [94, 95]. En ausencia de una diana, como lo indica la falta de una señal fluorescente, la sonda de ácido nucleico se hibrida y forma una estructura en bucle que acerca al fluoróforo y al extintor de sus extremos opuestos; mientras que la unión del analito a la sonda de ácido nucleico desplaza el fluoróforo de las AuNP, lo que da como resultado una señal fluorescente [24, 94, 96]. Aprovechando las propiedades mencionadas anteriormente, se incorporaron AuNP en balizas moleculares para la detección in vitro (nanoesferas de oro, AuNS) e in vivo (nanobarras de oro, AuNR) de la expresión de matriptasa en células tumorales. The two molecular beacons were composed of a matriptase cleavage site as a linker between the AuNPs and the fluorophores. The AuNS–molecular beacon was constructed with the fluorescein isothiocyanate (FITC), and the AuNR–molecular beacon had a NIR fluorescent dye (mercaptopropionic acid, MPA). In the absence of the target, the AuNSs and AuNRs, respectively, blocked the FITC and MPA fluorescence. Cleavage of either FITC or MPA from the AuNP–molecular beacons in the presence of matriptase exhibited a quantifiable fluorescence signal. The fluorescent signal of the MPA–AuNR–beacon in the nude mice bearing HT-29 tumors lasted for 14 h in the tumor site, while the signal gradually disappeared from the non-tumor site over time [97].
The AuNPs were reported to have comparable or higher fluorescence quenching efficiency than organic quenchers such as 4-((4′-(dimethyl-amino)phenyl)azo)benzoic acid (DABCYL) [94, 98] and Black Hole Quencher-2 [99]. The fluorescence quenching efficiency of 1.4 nm AuNPs was compatible with the four commonly used organic fluorophores (FITC, rhodamine, texas red and Cy5). The fluorescence quenching efficiency of the AuNPs was similar to that of DABCYL, and unlike DABCYL, the AuNPs showed consistency in both low and high salt buffers [98]. In a competitive hybridization assay, 10 nm AuNPs showed superior (> 80%) fluorescence quenching efficiency for Cy3 dye than the commercial Black Hole Quencher-2 (~ 50%). The assay had a limit of detection of 3.8 pM and a detection range coverage from 3.8 pM to 10 nM for miRNA-205 in human serum, and it was able to discriminate between miRNAs with variations in their nucleotide sequence [99]. The competitive sensor arrays were not only sensitive [96, 99] but were able to differentiate between normal and diseased cells, as well as benign and metastatic cancers [96].
AuNP-Based Bio-barcoding Assay
AuNP-based bio-barcoding assay (BCA) technology has become one of the highly specific and ultrasensitive methods for detection of target proteins and nucleic acids up to 5 orders of magnitude than the conventional assays [100]. The assay relies on magnetic microparticle probes, which are functionalized with antibodies that bind to a specific target, and AuNP probes encoded with DNA that recognizes the specific protein target and antibodies. Upon interaction with the target DNA, a sandwich complex between the magnetic microparticle and AuNPs probes is formed. The sandwich is then separated by the magnet followed by thermal dehybridization to release the free bar-code DNA, enabling detection and quantification of the target [101, 102].
The AuNP-based BCA assay was able to detect HIV-1 p24 antigen at levels that was 100–150-fold higher than the conventional ELISA [103]. The detection limit of PSA using these systems was 330 fg/mL [104]. The versatility of AuNPs for the development of a BCA-based platform was further demonstrated by measuring the concentration of amyloid-beta-derived diffusible ligands (ADDLs), a potential Alzheimer's disease (AD) marker found in the cerebrospinal fluid (CSF). ADDL concentrations were consistently higher in the CSF taken from the subjects diagnosed with AD than in non-demented age-matched controls [105]. These results indicate that the universal labeling technology can be improved through the use of AuNPs to provide a rapid and sensitive testing platform for laboratory research and clinical diagnosis.
AuNP-Based Therapies
Metal-based drugs are not new to medicine; in fact, they are inspired by the existing metallic drugs used in clinical treatment of various diseases [9, 106,107,108,109]. The widely studied and clinically used metal-based drugs were derived from platinum (e.g., cisplatin, carboplatin, tetraplatin for treatment of advanced cancers), bismuth (for the treatment of infectious and gastrointestinal diseases), gold (for the treatment of arthritis) and gallium (for the treatment of cancer-related hypercalcemia) [108, 109]. The approval of cisplatin in 1978 by the FDA for the clinical treatment of cancer [107] further inspired research on other metals (such as palladium, ruthenium, rhodium) [32, 106, 110].
Owing to the bioactivities, which included anti-rheumatic, antibacterial and anticancer effects, and the biocompatibility of bulk gold [8, 9, 46, 111], AuNPs are extensively investigated for the treatment of several diseases. AuNPs displayed unique and novel properties that are superior to its bulk counterpart. AuNPs are highly stable and have a distinct SPR, which guides their application in medicine [112], as drug delivery and therapeutic agents. AuNPs have a lot of advantages over the conventional therapy; they have a longer shelf-life and can circulate long enough in the system to reach their targets [25] with [11, 49, 113] or without targeting molecules [14, 15, 24, 25, 114]. AuNPs can provide localized and selective therapeutic effects; some of the areas in which AuNPs were used in therapy are described below.
Therapeutic Effects of Untargeted AuNPs
The as-synthesized (i.e., unmodified or uncapped) AuNPs have been shown to have diverse therapeutic effects against a number of infectious [115, 116], metabolic and chronic diseases [3, 29, 50, 51]. Their antioxidant, anticancer, anti-angiogenic [3, 32], anti-inflammatory [3, 51] and weight loss [29, 50, 112] effects are beneficial for diseases such as cancer, rheumatoid arthritis, macular degeneration and obesity [5, 25, 113, 117]. The above-mentioned diseases are characterized by a leaky vasculature and highly vascularized blood vessels [5, 113], which provides the NPs an easy passage into the diseased tissues and increase the susceptibility of cells to their effects. Through the EPR effect, uncapped AuNPs can passively accumulate in the vasculature of diseased cells or tissues. Hence, AuNPs have been specifically designed to have anti-angiogenic effects in diseases where angiogenesis (the growth and extension of blood vessels from pre-existing blood vessels) spins out of control like cancer, rheumatoid arthritis, macular degeneration and obesity [5, 25, 113, 117]. Targeting and destroying the defective blood vessels prevent oxygen and nutrients from reaching the diseased cells, which results in their death. The pores in the blood vessels at the diseased site (especially in cancer and obesity) are 200–400 nm and can allow materials in this size range to pass from the vasculature into the diseased tissues and cells [14, 15, 25, 114].
The cellular uptake, localization, biodistribution, circulation and pharmacokinetics of the uncapped AuNPs rely strongly on size and shape [49]. Although these effects are applicable to all AuNPs, the biological effects of citrate-capped AuNPs (cAuNPs) are extensively studied and reviewed. Spherical cAuNPs demonstrated selective in vitro anticancer activity that was size and concentration dependent on murine and human cell lines [3, 51]. Different sizes (10, 20 and 30 nm) of cAuNPs showed differential effects in human cervical carcinoma (HeLa), murine fibroblasts (NIH3T3) and murine melanoma (B16F10) cells. The 20 and 30 nm cAuNPs showed a significant cell death in HeLa cells starting at the lowest concentration of 2.2 µg/mL, while the 10-nm NPs was toxic at concentrations ≥ 8.75 µg/mL. The activity of these NPs was negligible in the noncancerous NIH3T3 cells, especially the 10 and 20 nm. The 20 nm reduced viability by ≤ 5% at the highest concentration (35 µg/mL), and ~ 20% for the 10 and 30 nm. The IC50 values for 10, 20 and 30 nm cAuNPs in the Hela cells were 35, 2.2 and 4.4 μg/mL, respectively, while the IC50 values for noncancerous cells were higher than 35 µg/mL [3]. Using a concentration range of 0.002–2 nM, 13 nm cAuNPs induced apoptosis in rabbit articular chondrocytes and no effects were observed for 3 and 45 nm cAuNPs under the same conditions. The 13 nm cAuNPs induced mitochondrial damage and increased reactive oxygen species (ROS); these actions could not be blocked by pre-treatment with a ROS scavenger, the N-acetyl cysteine [51]. Size-dependent effects were also observed in vivo after injecting cAuNPs of various sizes (3, 5, 8, 12, 17, 37, 50 and 100 nm) into mice (8 mg/kg/week) for 4 weeks. The 8, 17, 12 and 37 nm were lethal to the mice and resulted in tissue damage and death after 14 days of treatment; the other sizes were not toxic and the mice survived the experimentation period. On the contrary, the same-size AuNPs at a concentrations up to 0.4 mM were not toxic to HeLa cells after 24 h exposure [118].
The cAuNPs can interact and accumulate nonspecifically within various tissues and organs in the body, especially in the reticuloendothelial system (RES) organs (blood, liver, spleen, lungs) [55, 119]. This was evident in high-fat (HF) diet-induced obese Wistar rats [55] and Sprague–Dawley rats [119] following acute (1 dose for 24 h) [55] and chronic (1 dose; 0.9, 9 and 90 µg/week over 7 week period) [119] exposure to 14 nm cAuNPs, respectively. Majority of the i.v injected cAuNPs were detected in the liver, spleen, pancreas, lungs, kidneys [55, 119] including the skeleton and carcass of the rats [119]. Chen et al. observed that after intraperitoneal (i.p) injection of a single dose (7.85 µg/g bodyweight) of 21 nm cAuNPs in lean C57BL/6 mice, they accumulated in the abdominal fat tissues and liver after 24–72 h [29], as well as the spleen, kidney, brain and heart in the HF-induced obese mice that were injected with the same dose daily for 9 weeks [50]. The cAuNPs reduced the abdominal WATs (retroperitoneal and mesenteric) mass and blood glucose levels 72 h post-injection [29]. In the diet-induced obese mice, the 21 nm cAuNPs demonstrated anti-inflammatory and anti-obesity effects [50]. They also improved glucose tolerance, enhanced the expression of inflammatory and metabolic markers in the retroperitoneal WATs and liver [50]. Both the 14 and 21 nm cAuNPs showed no sign of toxicity or changes in the markers associated with kidney and liver damage [29, 55, 119].
Similar findings were reported for plant-mediated AuNPs, without targeting molecules they can access, ablate tumors [40, 120] and obese WATs [121] in rodents. Differential uptake, distribution and activity of biogenic AuNPs also vary depending on the size and shape of the NPs. While certain sizes can pass through the vascular network and be retained at the site of the disease; others can be easily filtered out of the system through the RES organs and the mononuclear phagocytic system as shown in Fig. 6 [15, 114]. NPs can be removed by tissue-resident macrophages (TRMs) before they reach the disease cells. Those that escape the TRMs and do not reach the disease site, especially smaller NPs (≤ 5 nm), are excreted through glomerular filtration in the kidney [25, 114]. Pre-treatment with clodronate liposomes depleted the TRMs in the liver and spleen before exposure to 50, 100 and 200 nm AuNPs. This reduced uptake of the AuNPs by the liver, increased their half-life in the blood as well as their accumulation at the tumor site [122]. However, TRMs are not the only obstacle that the AuNPs that rely on EPR effect for uptake must overcome. EPR effect alone can only ascertain ≤ 1% AuNP uptake [15, 114], and depletion of the TRMs prior to treatment resulted in just ≤ 2% of NPs reaching the target [122]. The success of non-targeted AuNPs depends on their ability to reach and accumulate in the diseased tissues, of which passive targeting through the EPR effect might not be efficient. The NPs also need to circulate longer, escape early clearance, and most importantly show reduced bystander effects [25, 123]. These qualities can increase bioavailability and ensure selectivity and efficacy of the AuNPs. These can further be improved by changing the surface chemistry of the AuNPs as discussed below [15, 124].
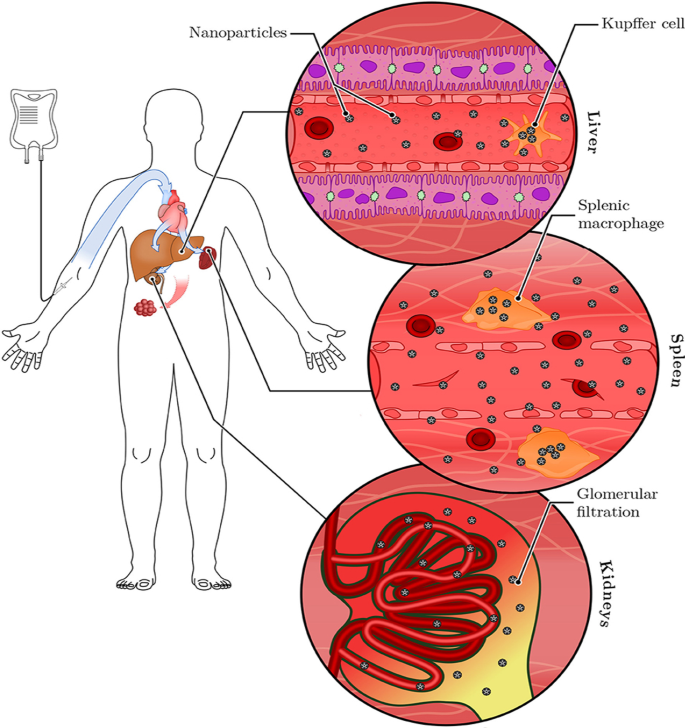
RES-based clearance of systemic administered AuNPs depends on their size. Large AuNPs accumulate in the liver, while smaller AuNPs are likely to end up in the spleen or be excreted in the urine via glomerular filtration. The AuNPs that escape the TRMs could accumulate in the diseased tissues. Reproduced with permission [114]. Copyright 2019, Frontiers in Bioengineering and Biotechnology
Therapeutic Effects of Surface-Functionalized AuNPs
The common strategy in AuNP-based therapeutics involves modifying the AuNP surface with therapeutic agents [3, 124,125,126]. The therapeutic agents can be drugs already used for the treatment of a particular disease or biomolecules with known inhibitory effects on cell signaling. In some instances, the therapeutic AuNPs have also been designed to have molecules that facilitate active targeting of the AuNPs toward specific cells and tissues. The molecules can easily adsorb on the AuNP surface by thiolation, chemical modification using chemistries such as 1-ethyl-3-(3-dimethylaminopropyl) carbodiimide (EDC), streptavidin/biotin binding [3, 124,125,126,127] and ionic interactions based on opposite charges between the NP surface and the biomolecules [124,125,126]. Functionalization of the AuNP surface influences their physicochemical properties and can affect their safety, biocompatibility and mobility. To ensure that the cargo carried by the AuNPs is delivered to the intended site, consideration should thus be given to both the physical and chemical properties of the AuNPs [124,125,126]. It is especially the size, shape, charge and the capping agents of the AuNPs that play an important role in the functionality of the AuNP conjugates [124] and can completely alter the pharmacokinetics of the AuNP-based therapeutics.
Functionalization allows for the development of customized nanosystems to reduce undesirable bystander effects often associated with traditional medicine. Functionalization of AuNPs can also prevent nonspecific adsorption of proteins onto the AuNP surface which can result in the formation a protein corona, resulting in the early clearance of the AuNPs through opsonization by the phagocytic cells [49, 123]. The surface charge of NPs can have a major influence on the behavior of NPs within biological environments. AuNPs with a neutral surface charge are unreactive and have a higher rate of escaping opsonization than charged AuNPs. Hydrophilic NPs will also behave differently to those with hydrophobic surfaces [49, 123]. PEG is one of the polymers most often used to mask AuNPs from phagocytic cells and has been shown to stabilize and enhance the biocompatibility of the AuNPs in numerous in vivo studies [49, 55, 123]. Pegylation improved the biocompatibility of 8.2 nm AuNPs by preventing neutrally and negatively charged AuNPs to bind to cell membranes or localize to any cellular components in African green monkey kidney (COS-1) cells [49]. And when the pegylated AuNPs were functionalized with a polyarginine cell penetrating moiety, the AuNPs were visualized on the cell membrane and inside the COS-1 cells [49]. Cell-penetrating peptides such as nuclear localization signal from SV40 virus, Tat from HIV and polyarginine peptides have been explored in translocation of AuNPs inside all cell type, normal or diseased. However, high specificity is required for clinical applications and can be achieved by taking advantage of the physiological differences between malignant and normal cells. This has been achieved by functionalizing the AuNPs with targeting molecules that recognize cell-specific receptors that are exclusively or overexpressed on the surface of target cells. This way, the AuNPs can be directed and delivered only to cells that express the target receptor. Therefore, conjugation of targeting moieties to the AuNPs (active targeting) will provide more selectivity, reduced bystander toxicity and enhanced efficacy since the AuNPs will be confined only to malignant tissues that express the target receptors [49, 55, 57, 113, 126, 127].
A good example to demonstrate the versatility of AuNPs is shown in Fig. 7, where four different molecules were conjugated onto the AuNPs to target two independent markers and mechanisms [11]. The multifunctional AuNPs were used for the treatment of leukemia (K562DR) cells that are resistant to doxorubicin (Dox). The 40 nm AuNPs were modified with two targeting moieties (folate and AS1411 aptamer) and two therapeutic agents (Dox and anti-miRNA molecules/anti-221). Folate molecule and AS1411 aptamer, respectively, recognize the folate and NCL receptors that are overexpressed on the cell surface and through receptor-mediated endocytosis will traffic the AuNP-conjugate into the cells. The AS1411 aptamer had dual functions, by also targeting the NCL receptor that is expressed inside the cells. After the AuNP-conjugate has been shuttled into the cells, the cargo (AS1411 aptamer, anti-221 and Dox) is off-loaded which independently act on three mechanisms that will synergistically bring about the demise of the cells. AS1411 aptamer together with anti-221 prevented leukemogenesis by suppressing the endogenous NCL and miR-221 function in the NCL/miR-221 pathway, thereby sensitizing the cells to the effects of Dox [11].
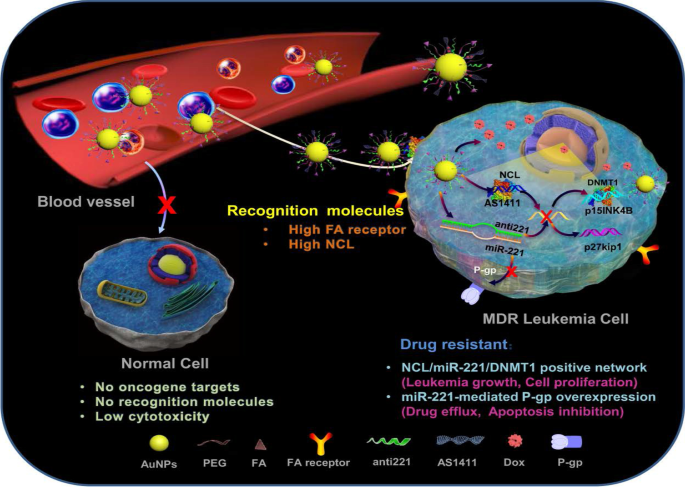
Multifunctional AuNPs in the treatment of multidrug-resistant (MDR) leukemia cells by increasing the sensitivity of the cells to Dox. Reproduced with permission [11]. Copyright 2019. Springer Nature. Folate (FA) receptor
Interestingly, similar dual targeting and treatment effects were achieved with green synthesized AuNPs without any additional molecules. With natural products acting as reducing agents, the biogenic AuNPs might also be more biocompatible than the chemically synthesized NPs [12, 40, 41, 43, 120]. MGF-AuNPs selectively targeted the laminin receptors in prostate (PC-3) and triple-negative breast cancer (MDA-MB-231) cells, and their xenografts in severe combined immunodeficiency (SCID) mice bearing these tumors [12, 40, 120]. In the normal SCID mice, the majority (85% at 30 min increasing to 95% after 24 h) of the i.v-injected MGF-AuNPs accumulated in the liver. Less than 10% were detected in the blood (2.7%), spleen (5%), lungs (0.6%), stomach, intestines and kidneys. When intra-tumorally injected in SCID mice-bearing prostate tumors, only 11% of the MGF-AuNPs were detected in the liver 24 h post-injection, while ~ 80% was in the tumor. Negligible amounts were found in the stomach, carcass and the small intestines. Some of the AuNPs were excreted through the renal and hepatic pathways in the urine and feces after 24 h [40, 120]. Nano Swarna Bhasma, a mixture consisting of AuNPs synthesized from mango peel extracts and phytochemicals from mango, turmeric, gooseberry and gum arabic, showed reduced toxicity toward normal endothelial cells after 48 h compared to the MDA-MB-231 cells [12].
Several studies have demonstrated that AuNPs have potential for clinical application. In combination with conventional drugs, it can be used to sensitize diseased cells to the drug effects [12, 128] and also prevent or reduce drug-related bystander effects [12]. AuNPs improved the pharmacokinetics of chemotherapeutic drugs, such as Dox [43, 129] and 5-fluorouracil (5-FU) [128]. Great improvements were mostly seen in the permeability and retention of drugs in the diseased cells, resulting in enhanced efficacy [130]. Dox-loaded AuNPs, which were non-toxic toward normal mouse fibroblast (L929) cells, also demonstrated selective toxicity toward fibrosarcoma tumors in mice [129]. 5-FU conjugated to the cAuNPs had better activity than 5-FU on its own in colorectal cancer cells [128]. AuNP co-treatment with chemotherapeutic drugs was highly efficient in improving the efficacy of chemotherapeutic drugs [12, 43, 128, 129, 131]. Orally ingested Nano Swarna Bhasma in combination with Dox and Cyclophosphamide reduced tumor volumes in SCID mice-bearing breast tumor cells and also showed acceptable safety profile and reduced bystander effects of the chemotherapeutic drugs in stage IIIA/B metastatic breast cancer patients [12]. Active targeting alone can ensure that the AuNPs are directly delivered into the desired targets, achieving a balance between efficacy and toxicity while minimizing damage to healthy tissues [14, 15, 49]. Controlled drug release is also among the many advantages offered by the AuNP-based systems and is crucial as it allows for localized and selective toxicity [49]. The AuNPs can be designed in such a way that their conjugates respond to internal (glutathione displacement, enzyme cleavable linkers, pH) or external (light, heat) stimuli to function [24, 25, 34, 128].
AuNPs as Transfection Agents in Gene Therapy
The use of AuNPs in gene therapy has shown promising outcomes by facilitating the delivery of genetic material to cells to silence or enhance expression of specific genes [24, 32, 132]. Thus, AuNPs can be used as transfection reagents in gene therapy for the treatment of cancer and other genetic disorders. AuNP conjugates have demonstrated higher transfection efficiency than experimental viral and non-viral gene-delivery vectors including polycationic reagents that has been approved for clinical use [24].
AuNPs are highly conductive and well suited for use as microelectrodes during electroporation for intracellular delivery of biomolecules for disease treatment. AuNPs significantly enhanced the performance of electroporation systems and have been used successfully for the delivery of DNA into hard-to-transfect cells such as the K562 cells [133]. To prevent cell loss which is often associated with electroporation, targeting moieties can be conjugated to the AuNPs to facilitate cellular uptake of AuNP conjugates through receptor-mediated mechanisms [133]. The use of AuNPs to transfect cells with oligonucleotide molecules also has the added advantage of increasing the half-life of these biomolecules and their efficacy [24, 32].
Untargeted AuNP conjugates are passively transported into cells and rely on the surface charge and AuNP shape for efficient transfection [24, 36, 134, 135]. The charge of the biomolecules that are conjugated onto AuNP surface plays a crucial role in their transfection efficiency; for instance, AuNPs functionalized with cationic molecules produce higher transfection efficiency than AuNPs functionalized with anionic molecules. Positively charged amino acids (lysine) can be attached on the NP surface to increase the rate of transfection. AuNSs [24] and AuNRs [36, 134, 135] are commonly used for transfections, and relative to the conventional transfection reagents (X-tremeGENE and siPORT), they inhibited the expression of target gene by > 70% in vitro [134] and in vivo [135]. In these studies, transfection efficiency was quantified based on target expression using RT-PCR and immunostaining [134, 135]. As transfection reagents, AuNPs provide long-lasting effects, localized gene delivery and higher efficacy [36, 134, 135]. Other types of nanomaterials (e.g., polymeric, liposomes, ceramic and carbon nanotubes) had received more attention for use in gene therapy than AuNPs. Six clinical trials using either polymeric or lipid-based nanomaterials for delivery of siRNA in solid tumors have been completed [36, 134, 136]. All of which suffer from low loading efficiency, low stability, and insufficient payload release [36, 136]. On the other hand, transfection systems based on AuNPs make use of easy chemistry that ensures efficient loading capacity and formation of stable complexes [36, 135]. Their safety can be controlled by manipulating their shape, size distribution and surface composition [36].
Antimicrobial Effects of AuNPs
MDR microbes are a major health concern and a leading cause of mortality, worldwide [21, 137,138,139,140,141]. These microorganisms have become resistant to conventional antimicrobial agents, due to over-prescription and misuse of these drugs [142]. No new antibiotics have been produced in over 40 years, mainly because the big pharmaceutical companies have retreated from their antibiotic research programs due to the lack of incentives [143]. As such, new and effective antimicrobial agents are urgently required to combat what could be the next pandemic, the antimicrobial resistance, and avoid surge in drug-resistant infections.
AuNPs are among the new generation of antimicrobial agents under review. They have shown broad antimicrobial (bactericidal, fungicidal and virucidal) effects against a number of pathogenic and MDR microorganisms and thus have potential to overcome microbial drug resistance [21, 142, 144]. Their antimicrobial effects are dependent on their physicochemical properties, especially their size, surface composition, charge and shape [21, 144]. Due to their small size, AuNPs can easily pass through the bacterial cell membrane, disrupt their physiological functions and induce cell death [35]. The exact antimicrobial mechanisms of AuNPs are not yet fully elucidated; despite this, some of the reported modes of actions that results from the interaction of various nanostructured materials (NSMs) with the bacterial cells are illustrated in Fig. 8. The highlighted mechanisms are also implicated in antimicrobial activity of AuNPs, they include induction of microbial death through membrane damage, generation of ROS and oxidative stress, organelle dysfunction, and alteration of gene expression and cell signaling [141].
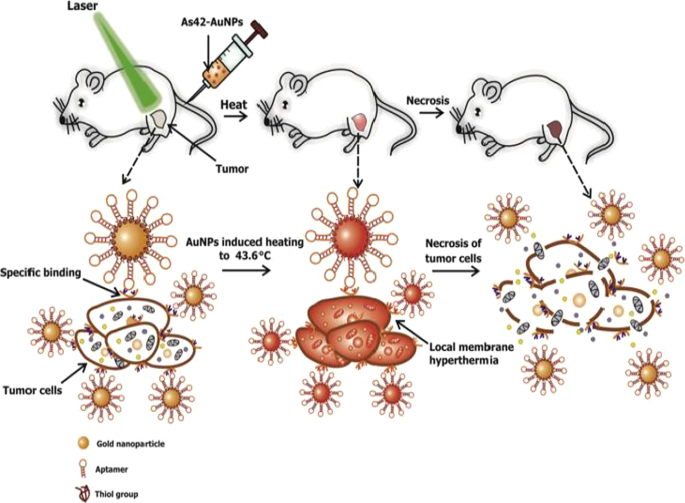
Antimicrobial mode of actions of the NSMs. Various NSMs can induce cell death by altering various biological functions, X represents alteration of cell signaling by de-phosphorylation of tyrosine residues in proteins as one of the mechanisms. Reproduced with permission [141]. Copyright 2018, Frontiers in Microbiology
AuNPs have multiple roles to play toward the development of antimicrobial agents, aside from being antimicrobial agents by themselves; they can serve as drug sensitizers and drug delivery vehicles [35, 58, 132, 145]. These features are applicable to both the chemical and green synthesized AuNPs, which have been reported to have antimicrobial effects against a number of human [21, 145,146,147] and waterborne [148] pathogenic strains. Generally, the test bacteria had shown low susceptibility toward the chemically synthesized AuNPs, i.e., the cAuNPs [21, 146, 147] and the NaBH4 -reduced AuNPs [149]. This was due to the repulsive forces between the negative charges on the AuNP surfaces and bacterial cells, thus preventing the interaction between AuNPs and the bacteria [21]. The activity of chemically synthesized AuNPs is based on their size, shape, concentration and exposure time. As an example, one study reported that NaBH4 -reduced AuNPs had no activity against Staphylococcus aureus (S. aureus ) and Escherichia coli (E. coli ) at 500 µg/mL for the duration of 6 h [149]. In contrast, another study showed a significant dose (1.35, 2.03 and 2.7 μg/mL) and size (6–34 nm vs 20–40 nm) dependent antibacterial effects of the NaBH4 -reduced AuNPs on Klebsiella pneumonia , E. coli , S. aureus and Bacillus subtilis [145].
The AuNPs are either used alone or in combination with other antimicrobial agents to treat microbial infections [35, 58, 132, 145]. When used in combination with other antimicrobial agents, the AuNP conjugates resulted in synergistic antimicrobial effects that surpassed the individual effects of the AuNPs and drugs [21, 35, 58, 132, 150]. These drugs were conjugated onto the AuNPs by either chemical methods [4, 151] or the drugs were used as reducing and capping agents [21, 149]. By so doing, the AuNPs improved drug delivery, uptake, sensitivity and efficacy. Some of the FDA-approved antibiotics and non-antibiotic drugs that were loaded onto the AuNPs are shown in Table 1 [4, 21, 149, 152]. Ciprofloxacin [152], cefaclor [149], lincomycin [4], kanamycin [21], vancomycin, ampicillin [151] and rifampicin [32] are among the antibiotics loaded on the AuNPs and demonstrated the versatility of AuNPs. These strategies were successful with various sizes and shapes of AuNPs, including gold silica nanoshells [152], AuNP-assembled rosette nanotubes [151] and AuNPs encapsulated in multi-block copolymers [153]. For instance, cefaclor-reduced AuNSs inhibited the growth of S. aureus and E. coli within 2–6 h depending on the concentration (10–50 µg/mL), while complete bacterial growth inhibition by the drug alone was only observed at 50 µg/mL after 6 h. The minimum inhibitory concentration (MIC) of the treatments was 10 µg/mL and 50 µg/mL for cefaclor-AuNPs and cefaclor, respectively [149].
AuNPs have presented properties that make them ideal candidates as alternative antimicrobial agents; the most important being their broad antimicrobial activity [21, 35, 58, 132, 150]. Owing to their biocompatibility and easily modifiable surface, microorganisms are less prone to developing resistance toward AuNPs [21]. For example, the kanamycin (Kan)-resistant bacteria (S . bovis , S . epidermidis , E . aerogenes , P . aeruginosa and Y . pestis ) showed increased susceptibility toward Kan-reduced AuNPs. The MIC values for Kan-AuNPs on the test bacteria were significantly reduced to < 10 µg/mL when compared to the MIC values for Kan alone at 50–512 µg/mL. This shows that AuNPs can restore the potency of antibiotics toward the drug-resistant strains by facilitating the uptake and delivery of the antimicrobial agents [21]. AuNPs can enhance drug-loading capacity and control the rate at which the drugs are released. AuNP hybrids with the multi-block copolymers increased the loading capacity of rifampicin and the drug’s half-life to 240 h. By sustaining the drug in the system for that long, ensured slow release of rifampicin from AuNPs at the target sites after oral administration of the AuNP conjugates to rats for 15 days. The drug on the surface was released within 24 h followed by the drug trapped in the polymer matrix after 100 h. And lastly, the drug entrapped between the AuNPs and the polymer matrix took over 240 h to be released in the interstitial space [153].
The AuNP hybrids also allow for the conjugation of multiple molecules with independent but synergistic functions. This was demonstrated by co-functionalization of the AuNPs with antimicrobial peptide (LL37) and the pcDNA that encode for pro-angiogenic factor (vascular endothelial growth factor, VEGF) and used in the treatment of MRSA-infected diabetic wounds in mice [132]. The AuNPs served dual functions, as a vehicle for the biomolecules, and also as transfection agent for the pcDNA. After topical application of the AuNP conjugates on the wound, the LL37 reduced MRSA colonies, while the pcDNA promoted wound healing by inducing angiogenesis through the expression of VEGF [132].
AuNPs have been shown to confer activity and repurpose some non-antibiotic drugs toward antimicrobial activity. The examples of repurposed drugs, which were used for the treatment of diseases other than bacterial infections, include 5FU [58], metformin [147] and 4,6-diamino-2-pyrimidinethiol (DAPT) [13, 112]. AuNPs as drug carriers are able to transport the drugs into the cells and allow direct contact with cellular organelles that resulted in their death [58, 147]. 5FU is an anti-leukemic drug, when attached to AuNPs was shown to kill some bacterial (Micrococcus luteus , S. aureus , P. aeruginosa , E. coli ) and fungal (Aspergillus fumigatus , Aspergillus niger ) strains [58]. While bacteria are resistant to DAPT, DAPT-AuNPs displayed differential antibacterial activity against the Gram-negative bacteria. Furthermore, conjugation of non-antibiotic drugs (e.g., guanidine, metformin, 1-(3-chlorophenyl)biguanide, chloroquine diphosphate, acetylcholine chloride, and melamine) as co-ligands with DAPT on AuNPs exerted non-selective antibacterial activity and a two–fourfold increased activity against Gram-negative bacteria [13]. When used in vivo, orally ingested DAPT-AuNPs showed better protection by increasing the intestinal microflora in E. coli -infected mice. After 4 weeks of treatment, the DAPT-AuNPs cleared the E. coli infection with no sign of mitochondrial damage, inflammation (increase in firmicutes ) or metabolic disorders (reduction in bacteroidetes ) in the mice [112].
The virucidal effects of the AuNP-based systems have been reported against several infectious diseases caused by influenza, measles [154], dengue [155, 156] and human immunodeficiency [115] viruses. Their anti-viral activity was attributed to the ability of AuNPs to either deliver anti-viral agents, or the ability to transform inactive molecules into virucidal agents [154, 156]. AuNPs synthesized using garlic water extracts inhibited measles viral growth in Vero cells infected with the measles virus. When the cells were exposed to both the virus and AuNPs at the same time, they blocked infection of Vero cells by the measles virus [154]. The AuNPs were nontoxic to the Vero cells up to a concentration of 100 µg/mL but inhibited viral uptake by 50% within 15–30 min at a concentration of 8.8 μg/mL [154]. Based on the Plaque Formation Unit assay, the viral load was reduced by 92% after 6 h exposure to 8.8 μg/mL of the AuNPs. The AuNPs interacted with the virus directly and blocked its transmission into the cells [154]. Modification of the AuNP surface with ligands that bind to the virus [156] or anti-viral agents [115, 155] protected them from degradation, enhanced their uptake and delivery onto the cells. The charge of the AuNPs also played a role, with cationic AuNPs being more effective in the delivery and efficacy of the AuNPs than the anionic and neutrally charged NPs. Cationic AuNPs complexed with siRNA inhibited dengue virus-2 replication in dengue virus-2-infected Vero and HepG-2 cells and also the virus infection following pre-treatment of the virus with AuNPs [155]. Inactive molecules are transformed into highly potent anti-viral agents after conjugation to AuNPs. One such example is the transformation of SDC-1721 peptide, a derivative of TAK-779, which is an antagonist of CCR5 and CXCR3 receptors for HIV-1 strain. SDC-1721 has no activity against the HIV-1, but when conjugated to the AuNPs it inhibited HIV-1 infection of the human phytohemagglutinin-stimulated peripheral blood mononuclear cells. The inhibitory effects of SDC-1721-AuNPs were comparable to the TAK-779 [115].
AuNPs as PT Agents
Diseased cells are sensitive to temperatures above 40 °C; cancer cells in particular appear to be even more sensitive to these high temperatures. Studies have shown that high fevers in cancer patients either reduced the symptoms of cancer or completely eradicated the tumors as a result of erysipelas infections [33, 157, 158]. Historically, fevers induced by bacterial infections, hot desert sand bath, or hot baths were used to increase the body temperature in order to kill the cancer cells [157]. These findings gave birth to PT therapy (PTT), which is mostly used for the treatment of cancer. PTT makes use of organic photosensitizers (indocyanine green, phthalocyanine, heptamethine cyanine) that are irradiated by the external source to generate heat energy that will increase the temperature to 40–45 °C (hyperthermia) in the target cells. Hyperthermia then triggers a chain of events (such as cell lysis, denaturation of the genetic materials and proteins), resulting in the destruction of the diseased cells [57, 158,159,160].
The organic dyes are used alone, or in combination with chemotherapy and radiotherapy for enhanced efficacy [157, 160]. Ideally, the effects of the PT agents must be confined to target cells and display minimal bystander effects. However, the organic PT dyes have several limitations such as toxic bystander effects, susceptibility to photobleaching and biodegradation [159]. In recent years, AuNPs are being explored as alternative PT agents as they exhibit strong plasmonic PT properties, and depending on their shape, they can absorb visible or NIR light. Absorption of light in the NIR spectrum is an added advantage that can allow deep tissue PTT [158, 161, 162]. Unlike organic dyes, AuNPs operate in an optical window where the absorption of light by interfering biological PT agents such as hemoglobin, melanin, cytochromes and water is very low [158, 161, 162].
The practicality of AuNP-based PTT has been demonstrated through in vitro and in vivo studies [158, 162, 163]. When the AuNPs are exposed to light, they can convert the absorbed light energy into thermal energy within picoseconds [57, 158, 159], consequently activating cell death via necrosis or apoptosis in the target cells or tissues. AuNP-based hyperthermia in diseased cells has been reported to occur at half the amount of the energy required to kill normal cells, thus perceived to be safer and better PT agents than the conventional dyes [33, 160]. AuNPs can be easily modified to have localized and enhanced PT activity by targeting and accumulating in only diseased cells through either active or passive targeting. And since the tumor environment is already hypoxic, acidic, nutrient starved and have leaky vasculature, the tumors will be most sensitive to the AuNP-based hyperthermia than the surrounding healthy cells and tissues [33, 160].
AuNP-based PTT has been extensively studied [158, 161, 162] and established that AuNPs (e.g., AuNRs, nanocages and nanoshells) that absorb light in the NIR spectrum are best for in vivo and deep tissue PTT [161]. While the ones that absorb and emit light in the visible spectrum (AuNSs and hollow AuNPs) have been demonstrated to treat diseases that affect shallow tissues (up to a depth of 1 mm), which could be of benefit to superficial tumors [158, 161, 162], ocular surgery [164, 165], focal therapy and vocal cord surgery [158, 165]. Although the PTT effects of AuNSs are limited in vivo or for use in deep tissues, combination therapy or active targeting can be incorporated to facilitate target-specific effects [158, 161, 163]. The AuNPs in the combination therapy will serve dual functions as both drug sensitizer and a PT agent, and was shown to enhance anticancer effects of chemotherapeutic drugs [158, 162, 163]. AuNS-Dox combination demonstrated enhanced cancer cell death after laser exposure when compared to the individual effects of the AuNSs and Dox with and without laser treatment [158].
Active targeting on its own can also improve AuNP uptake, localization and target-specific PT effects, which can be viewed in real time by adding fluorophores. AuNSs (25 nm) loaded with transferrin targeting molecules and FITC were shown to accumulate and destroy human breast cancer cells at a higher rate than in non-cancer cells and had better efficacy than the untargeted AuNSs [57]. An independent study also demonstrated that DNA aptamers (As42)-loaded AuNSs (As42-AuNP) induced selective necrosis in Ehrlich carcinoma cells that express HSPA8 protein, a receptor for the aptamers. None of these effects were observed in blood and liver cells mixed with target cells, or cells treated with the AuNSs without laser treatment [163]. The PT effects of the As42-AuNP were replicated in mice transplanted with Ehrlich carcinoma cells in their right leg. As shown in Fig. 9, tail-vein injections of As42-AuNPs followed by laser irradiation resulted in targeted PT destruction of the cancer cells. The As42-AuNPs reduced tumor size in a time-dependent manner; cell death was attributed to increased temperature up to 46 °C at the tumor site. The tumor in mice treated with As42-AuNPs without laser treatment and the AuNPs conjugated with nonspecific DNA oligonucleotide continued to grow but at the lower rate compared to mice injected with PBS. This suggests that the AuNPs were also localized in the tumor [163]. In cases where AuNSs are not efficient for deep tissue PTT, other shapes such as nanocages, nanoshells and AuNRs can be used [158]. Alternately, the visible light absorption of the AuNSs can be shifted to NIR by using processes such as two-photon excitation [57].
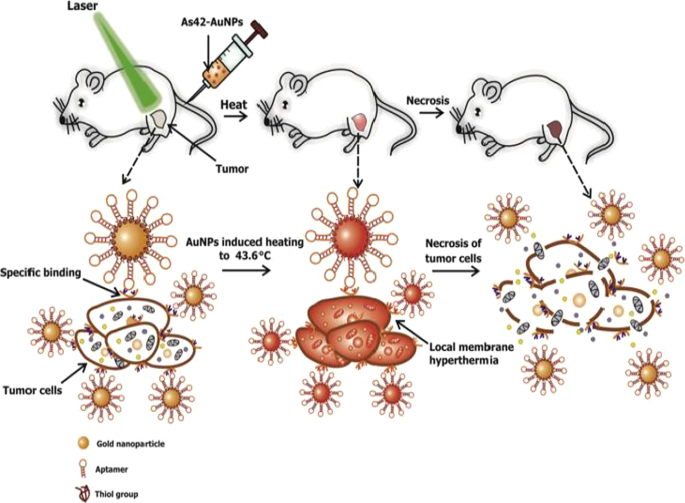
In vivo plasmonic PT therapy of cancer cells using targeted AuNSs. As42-AuNPs localized in HSPA8-expressing tumor cells after i.v injection. Exposure to laser treatment resulted in hyperthermia that caused cancer cell death. Reproduced with permission [163]. Copyright 2017, Elsevier
The PT effects of the AuNPs have also been reported for the reversal of obesity [52, 56], using hollow AuNSs (HAuNSs) [52] and AuNRs [56] for the PT lipolysis of the subcutaneous white adipose tissue (sWAT) in obese animals. The HAuNSs were modified with hyaluronate and adipocyte targeting peptide (ATP) to produce HA–HAuNS–ATP conjugate [52]. Hyaluronate was used to ensure topical entry of the HA–HAuNS–ATP through the skin [52, 166], while ATP will recognize and bind to prohibitin once the HAuNSs are internalized. Prohibitin is a receptor that is differentially expressed by the endothelial cells found in the WAT vasculature of obese subjects [5, 52, 55]. The HA–HAuNS–ATP was topically applied in the abdominal region of the obese mice, and through hyaluronate were transdermally shuttled through the epidermis into the dermis where the ATP located the sWATs (Fig. 10) . Illumination of the target site with the NIR laser selectively induced PT lipolysis of the sWAT in the obese mice and reduced their body weight [52]. The AuNRs were used in the photothermolysis-assisted liposuction of the sWATs in Yucatan mini pigs. The untargeted PEG-coated AuNRs (termed NanoLipo) were injected in the sWATs through an incision, followed by laser illumination to heat up the sWATs, which was then aspirated using liposuction. The amount of fat removed from NanoLipo-treated porcine was more than the one removed with conventional suction-assisted lipectomy (SAL). NanoLipo-assisted fat removal had several advantages over the conventional SAL; it took less time (4 min) for liposuction compared to 10 min for SAL, the swelling in the treated site healed faster, and the weight loss effects lasted over 3 months post-liposuction [56].
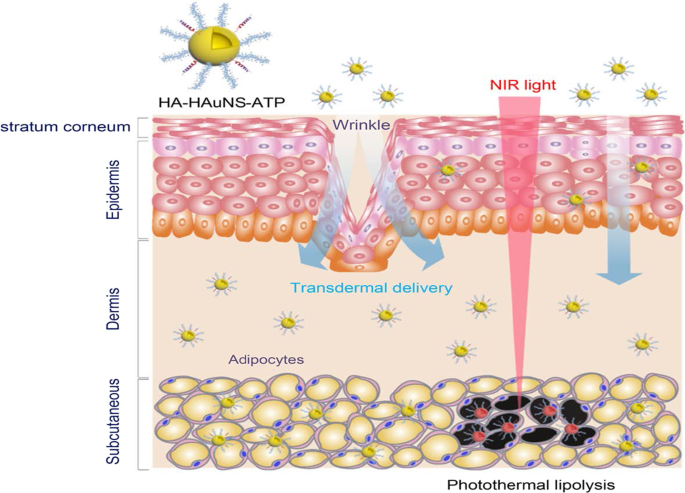
PT lipolysis of the sWATs using HA-HAuNS-ATP. The ATP was conjugated to the AuNSs for targeted delivery and destruction of the prohibitin-expressing sWATs after NIR laser exposure. Reproduced with permission [52]. Copyright 2017, American Chemical Society
AuNP-based PTT clearly offers a lot of advantages compared to the conventional agents. Their biocompatibility allows for broader applications both in vitro and in vivo. Moreover, they can be customized based on their shapes for shallow (AuNSs) [158, 161, 162] or deep tissue (AuNRs and stars) PTT [158, 161]. At 1–100 nm diameter, AuNPs and its conjugates can circulate long enough to reach and accumulate in the target tissues, with or without targeting moieties [159, 167]. Active targeting can be used to ensure localized PT effects through various routes of administration and might be effective for solid and systemic diseases. AuNP-based PTT can also be used to sensitize cancer cells when administered in combination with chemotherapy, gene therapy and immunotherapy [159]. Therefore, AuNP-based PTT has potential for treatment of chronic diseases [161].
Toxicity of AuNPs
AuNPs can play an important role in medicine, as demonstrated by the preclinical and clinical studies under review. Their full potential in clinical application as both diagnostic and therapeutic agents can only be realized if they do not pose any health and environmental hazards. While their use in vitro appears to be inconsequential, in vivo application can be hampered by their potential toxicity, which could be detrimental to human health. A major concern with their clinical use is that AuNPs are non-biodegradable and their fate in biological systems has not been fully studied [5, 30]. Although AuNPs are considered to be bio-inert and compatible, their properties (size, shape, charge and composition) raise concerns as they can alter their pharmacokinetics when used in biological environment [27, 34, 118]. The toxicity of AuNPs of varying sizes and shapes has been demonstrated in animals [27, 118]. These NPs can accumulate in the RES organs where they induce damage.
AuNPs are 1–100 nm in diameter which makes them smaller than most of the cellular components. At these sizes, AuNPs can passively transverse cellular barriers and blood vessels by taking advantage of the EPR effect in pathological cells. AuNPs with smaller diameters (1–2 nm) can easily penetrate cell membranes and biologically important cellular organelles such as mitochondria and nuclei [7, 168]. Accumulation of AuNPs in these organelles induces irreversible damage that can cause cellular demise. On the contrary, AuNPs larger than 15 nm are restricted to the cytoplasmic spaces and unable to penetrate internal organelles [168]. These features are desirable for targeting pathological cells, however, AuNPs can also be taken up by healthy cells and alter their physiology [118]. Administration of AuNP-based therapeutics can be done via different routes (i.e., intranasal, oral, transdermal, i.p or i.v) and transported through blood vessels into different tissues and organs [34, 118]. They are able to pass through the blood brain barrier and the placental barrier [34]. Toxicity is size dependent, with certain sizes of AuNPs being well tolerated, while others could be lethal to healthy tissues. Unfunctionalized AuNSs at 8, 17, 12, 37 nm caused physical changes (i.e., change the fur color, loss of bodyweight, camel-like back and crooked spine) within 14 days of treatment (2 doses of 8 mg/kg/week) in rats [118]. Most (> 50%) of the rats died within 21 days (i.e., after 3 doses), and abnormalities in the RES organs (liver, lungs and spleen) were observed. On the contrary, mice treated with 3, 5, 50 and 100 nm AuNPs were not affected by the NPs and no adverse effects or death occurred throughout the duration (50 days) of the study [118]. In diet-induced obese rats that received i.v injections of 14 nm cAuNPs, the NPs were detected in various tissues after 24 h and were mostly confined to the RES organs [55].
The shape, charge and surface chemistry of AuNPs can influence their toxicity. These factors can determine how AuNPs will interact with the biological systems, their cellular uptake and effects on the cells. AuNSs are readily taken up by cells and proven to be less toxic than other shapes such as rods and stars. AuNP surfaces are charged and will influence how they interact and behave within a biological environment [169]. Cationic AuNPs are likely to be more toxic compared to neutral and anionic AuNPs, as their charge allows these NPs to easily interact with negatively charged cell membranes and biomolecules such as DNA. Both the positively and negatively charged AuNPs have been associated with mitochondrial stress, which was not observed with the neutrally charged AuNPs [34, 35].
The shell that forms on the surface of the AuNP core can also influence the functioning of the NPs. These are usually reducing and/ or stabilizing agents such as citrate and CTAB, and once subjected to a biological environment, these molecules can cause either the desorption or absorption of biomolecules found in the biological environment. This can result in the formation of a corona or cause the NPs to become unstable. Citrate- and CTAB-capped AuNPs are highly reactive, which can facilitate the attachment of biocompatible polymers such as PEG, polyvinyl-pyrrolidone, poly (acrylic acid), poly(allylamine hydrochloride), and polyvinyl-alcohol) or biomolecules such as albumin and glutathione to prevent the formation of AuNP-corona with serum proteins. These molecules serve as a stabilizing agent and form a protective layer that can mask the AuNPs from attacks by phagocytes [7, 29, 34, 170] and prevent off-target toxicity [7]. As discussed in “AuNP-Based Therapies” section, AuNPs can be functionalized with targeting and therapeutic agents to define their targets and effects [34].
In addition to their physicochemical properties, the dosage, exposure time and environmental settings also influence the activity of AuNPs. Lower doses and short-term exposure times might render AuNP as nontoxic, while increasing these parameters will lead to cytotoxic effects [34]. Moreover, in vitro studies do not always simulate in vivo studies. At times, AuNPs that seem to be nontoxic in cell culture-based experiments end up being toxic in animal experiments. Many factors could be responsible for these discrepancies [118], and some steps have been identified that can guarantee the safety of AuNPs in biomedical applications. The biocompatibility and target specificity of AuNPs can be improved by modifying the surface of the NPs. Attaching targeting moieties on the AuNPs can channel and restrict their effects to specific targets or pathological cells [5, 55, 127]. Modification of AuNP surface with bio-active peptides provides a platform for developing multifunctional AuNPs with enhanced specificity, efficacy and potentially sustainable effects [11, 127]. All of these effects will be instrumental in the design and development of AuNP-based systems for clinical applications.
Clinical Application of AuNPs
Nanotechnology has the potential to shape the future of healthcare systems and their outcomes. Its promise of creating highly sensitive and effective nanosystems for medicine has been realized with the introduction of organic nanoformulations for cancer treatment. These systems have already paved the way for nanomaterials into clinical applications:doxil and abraxane have been in the market for over two decades and demonstrated the potential of nanotechnology in medicine [1, 2]. More recently, this technology has been used for the development of the SARS-CoV-2 lipid NP-based vaccine to fight against the COVID-19 pandemic [171]. Inorganic nanosystems such as AuNPs offer many advantages over their organic counterparts, yet few of these systems are used clinically (Table 2) [19, 32].
While several AuNP-based drugs are some of the inorganic nanomaterial-based drugs that were tested in clinical trials, they are not progressing at the same rate as organic liposome-based nanodrugs. Aurimune (CYT-6091) and aurolase were the first of AuNP-based formulations to undergo human clinical trials for the treatment of solid tumors. CYT-6091 clinical trials started in 2005 for delivery of recombinant TNF-α as an anticancer therapy in late-stage pancreatic, breast, colon, melanoma, sarcoma and lung cancer patients. CYT-6091 consists of 27-nm cAuNPs loaded with TNF-α and thiolated PEG. The CYT-6091 nanodrug has achieved safety and targeted biologic response at the tumor site at a dose lower than that required for TNF-α alone [16, 17]. CYT-6091 is approved and yet to start phase II clinical trials in combination with chemotherapy. Based on phase II clinical trial strategy, several variants of CYT-6091 have been developed and tested in preclinical studies. All the nanosystems contain TNF-α with either chemotherapy (paclitaxel, dox and gemcitabine), immunotherapy (Interferon gamma) or apoptosis inducing agents attached to the 27 nm cAuNPs [14,15,16]. The AuNP conjugates preferentially accumulated in the tumor sites after systemic administration through the EPR effect and vascular targeting effects of the TNF-α. The AuNPs were not detected in the healthy tissues, and the anti-tumor effects of TNF-α were restricted to the tumor environment [14, 16, 19].
The first clinical trial for the PT treatment with AuroLase® for refractory and/or recurrent head and neck cancers was completed. Information on the outcome of this trial is still pending. The second trial is set to evaluate the effects of AuroLase® on primary and/or metastatic lung tumors in patients where the airway is obstructed [19]. The number of human trials based on AuNP-based formulation is increasing, covering the treatment of a wide range of medical conditions including skin, oral, heart and neurological diseases. AuNP-formulation (150 nm silica-gold nanoshells coated with PEG), which is similar to AuroLase®, was approved for PT treatment of moderate-to-severe inflammatory acne vulgaris. The nanoshells were topically applied on the acne area and transdermally delivered into the follicles and sebaceous ducts through low-frequency ultrasound or massage. Nanoshells applied through massage were effective in penetrating the shallow skin infundibulum (90%) and the sebaceous gland (20%), while the low-frequency ultrasound can penetrate both shallow and deep skin tissues. NIR laser treatment resulted in focal thermolysis of the sebaceous glands in the affected area and disappearance of the acne [18, 167]. The gold–silica nanoshells were well-tolerated, showed no systemic toxic effects with minor side effects (reddiness and swelling) at the treatment site [18]. AuNPs offer many health benefits based on their unique properties but at the same time have raised a lot of political and ethical issues, and resulted in termination of some clinical studies (NCT01436123).
Conclusion and Future Perspectives
Applications of AuNPs in biomedicine are endorsed by their unique physicochemical properties and have shown great promise as theranostic agents. The increasing interest in biomedical applications of AuNPs is further encouraged by the biocompatibility and medical history of bulk gold, which suggests that the gold core in AuNPs will essentially display similar or improved properties [3]. But at the same time their small size can infer unique properties that will completely change their pharmacokinetics [144]. The diverse biomedical applications of AuNPs in diagnostics and therapeutics herein discussed demonstrate their potential to serve as adjunct theranostic agents. They can be used as drug delivery, PTT, diagnostic and molecular imaging agents [12, 33, 128]. In time, and with better knowledge of mechanisms of action, more AuNP-based systems will obtain approval for clinical use. However, the excitement of these biomedical applications of AuNPs should unequivocally be balanced with testing and validation of their safety in living systems before any clinical applications.
In conclusion, more work needs to be done to taper the toxicity of AuNPs. This can be achieved by introducing biocompatible molecules on their surface [14, 15, 58, 159], and developing new and better synthesis methods, such as the use of green chemistry to produce biogenic NPs. All these developments may further broaden the applications of AuNPs in nanomedicine. AuNPs are non-biodegradable, and off-target distribution could result in chronic and lethal effects. All these concerns must be addressed before clinical translation; the existing trials will soon provide some clarity on their impact in human health. Should their health benefits outweigh their potential risks as is the case with the existing clinical drugs, it is a matter of time before they are approved for clinical use.
Availability of Data and Materials
All the information in this paper was obtained from the studies that are already published and referenced accordingly.
Abbreviations
- 5-FU:
-
5-Fluorouracil
- AD:
-
Alzheimer's disease
- ADDLs:
-
Amyloid-beta-derived diffusible ligands
- ASOs:
-
Antisense oligonucleotides
- AuNPs:
-
Gold nanoparticles
- AuNPsQ:
-
Quantum-sized AuNPs
- AuNRs:
-
Gold nanorods
- AuNSs:
-
Gold nanospheres
- BCA:
-
Bio-barcoding assay
- cAuNPs:
-
Citrate-capped AuNPs
- COVID-19:
-
Corona virus disease 2019
- CSF:
-
Cerebrospinal fluid
- CT:
-
Computed tomography
- CTAB:
-
Cetyltrimethylammonium bromide
- DABCYL:
-
4-((4′-(Dimethyl-amino)-phenyl)-azo)benzoic acid
- DAPT:
-
4,6-Diamino-2-pyrimidinethiol
- Dox:
-
Doxorubicin
- EDC:
-
1-Ethyl-3-(3-dimethylaminopropyl) carbodiimide
- EPR:
-
Enhanced permeability and retention
- FA:
-
Folate
- FDA:
-
Food and Drug Administration
- FITC:
-
Fluorescein isothiocyanate
- FRET:
-
Fluorescence resonance energy transfer
- HAuNSs:
-
Hollow AuNSs
- HF:
-
High-fat
- HU −1 :
-
Hounsfield unit
- LFAs:
-
Lateral flow assays
- LSPR:
-
Localized surface plasmon resonance
- MDR:
-
Multidrug resistant
- MGF:
-
Mangiferin
- MPA:
-
Mercaptopropionic acid
- MWPLP:
-
Microwave-induced plasma-in-liquid process
- NCL:
-
Nucleolin
- NIR:
-
Near-infrared
- NSMs:
-
Nanostructured materials
- P. jirovecii :
-
Pneumocystis jirovecii
- PEG:
-
Polyethylene glycol
- PSA:
-
Prostate-specific antigen
- PSMA:
-
Prostate-specific membrane antigen
- PT:
-
Photothermal
- PTT:
-
Photothermal therapy
- QDs:
-
Quantum dots
- RES:
-
Reticuloendothelial system
- ROS:
-
Reactive oxygen species
- SARS-CoV-2:
-
Severe acute respiratory syndrome-coronavirus-2
- SCID:
-
Severe combined immunodeficiency
- SPR:
-
Surface plasmon resonance
- sWAT:
-
Subcutaneous white adipose tissue
- TOAB:
-
Tetrabutylammonium bromide
- TRMs:
-
Tissue-resident macrophages
- VEGF:
-
Vascular endothelial growth factor
- WAT:
-
White adipose tissue
Nanomateriales
- Nanopartículas de oro para sensores de quimioterapia
- Nanopartículas para la terapia del cáncer:avances y desafíos actuales
- Avances y desafíos de los nanomateriales fluorescentes para síntesis y aplicaciones biomédicas
- Compuestos de grafeno y polímeros para aplicaciones de supercapacitores:una revisión
- La preparación de la nanoestructura yema-cáscara de Au @ TiO2 y sus aplicaciones para la degradación y detección del azul de metileno
- Poliglicerol hiperramificado modificado como dispersante para el control del tamaño y la estabilización de nanopartículas de oro en hidrocarburos
- Preparación de micromateriales híbridos de MnO2 recubiertos de PPy y su rendimiento cíclico mejorado como ánodo para baterías de iones de litio
- Fabricación, caracterización y citotoxicidad de nanopartículas de carbonato de calcio derivadas de concha de oro-berberecho conjugado de forma esférica para aplicaciones biomédicas
- Avances recientes en métodos sintéticos y aplicaciones de nanoestructuras de plata
- Saponinas Platycodon de Platycodi Radix (Platycodon grandiflorum) para la síntesis verde de nanopartículas de oro y plata
- Nanopartículas de oro de forma redonda:efecto del tamaño de partícula y la concentración sobre el crecimiento de la raíz de Arabidopsis thaliana