Avances y desafíos de los nanomateriales fluorescentes para síntesis y aplicaciones biomédicas
Resumen
Con el rápido desarrollo de la nanotecnología, en las últimas dos décadas han surgido nuevos tipos de nanomateriales fluorescentes (FNM). La escala nanométrica confiere a los FNM propiedades ópticas únicas que desempeñan un papel fundamental en sus aplicaciones en bioimágenes y detecciones dependientes de la fluorescencia. Sin embargo, dado que la baja selectividad y la baja eficiencia de fotoluminiscencia de los nanomateriales fluorescentes dificultan hasta cierto punto sus aplicaciones en la formación de imágenes y la detección, los científicos todavía están buscando sintetizar nuevos FNM con mejores propiedades. En esta revisión, se resume una variedad de nanopartículas fluorescentes que incluyen puntos cuánticos semiconductores, puntos de carbono, nanopartículas de carbono, nanotubos de carbono, nanomateriales basados en grafeno, nanopartículas de metales nobles, nanopartículas de sílice, fósforos y estructuras orgánicas. Destacamos los avances recientes de los últimos desarrollos en síntesis de FNMs y sus aplicaciones en el campo biomédico en los últimos años. Además, se han revisado y discutido las principales teorías, métodos y limitaciones de la síntesis y aplicaciones de las FNM. Además, los desafíos en síntesis y aplicaciones biomédicas también se resumen sistemáticamente. También se presentan las direcciones y perspectivas futuras de las FNM en aplicaciones clínicas.
Introducción
Los colorantes orgánicos convencionales han enfrentado algunas dificultades en su aplicación en biomedicina debido a sus defectos inherentes como la citotoxicidad y la mala biocompatibilidad [1]. Sin embargo, la aparición de nanomateriales fluorescentes muestra un gran potencial en la sustitución de los tintes orgánicos convencionales. Los científicos han invertido mucho tiempo y esfuerzo en la investigación de nanomateriales fluorescentes, y los logros relevantes en síntesis y aplicaciones son más que inspiradores.
La forma, el tamaño y la estructura de los nanomateriales fluorescentes determinan sus propiedades físicas y químicas, que influyen enormemente en su rendimiento. Por lo tanto, la síntesis controlable de nanomateriales fluorescentes se ha convertido en un tema de investigación candente. Las condiciones experimentales óptimas de síntesis contribuyen al tamaño, morfología y estabilidad más adecuados de los nanomateriales fluorescentes. En los últimos años, se ha realizado un gran esfuerzo para mejorar la biocompatibilidad de los nanomateriales fluorescentes mejorando los métodos de síntesis [2]. Los iones metálicos generalmente se dopaban con puntos de carbono (CD) o puntos cuánticos (QD) para funcionalizar la superficie de los nanomateriales fluorescentes en el pasado. Sin embargo, la fluorescencia ineficaz y la toxicidad subyacente plantearon una amenaza para sus aplicaciones en bioimagen y bioetiquetado [3]. Teniendo en cuenta estos problemas, Zuo et al. informó de un sistema de entrega de genes CD de alta eficiencia. Los CD dopados con flúor se sintetizaron mediante un proceso solvotermal, y la polietilenimina ramificada (b-PEI) puede proporcionar sitios de carga positiva para la entrega de genes [4]. Se puede anticipar que los nuevos métodos de modificación de la superficie serán un área de investigación de puntos críticos en el futuro.
Se han realizado muchos esfuerzos para explorar el potencial de los nanomateriales fluorescentes para aplicaciones biomédicas que incluyen bioimagen, biodetección y algunos métodos de terapia, como se muestra en la Fig. 1. La fluorescencia confiable para su aplicación depende de sus propiedades físicas y químicas [5]. Por lo tanto, el trabajo de investigación para mejorar sus propiedades, como la toxicidad, la hidrofilicidad y la biocompatibilidad, ha sido una parte importante de la realización del uso extensivo de nanomateriales fluorescentes en áreas biomédicas. Con el aumento de la tasa de algunas enfermedades como el cáncer, existe una demanda creciente de estrategias novedosas de diagnóstico y terapia con mayor precisión y cumplimiento por parte de los pacientes [6]. Actualmente, el dopaje con iones metálicos o no metálicos y la modificación de la superficie de los nanomateriales fluorescentes siguen siendo las técnicas dominantes para mejorar su eficiencia PL y biocompatibilidad [7], y las investigaciones correspondientes abren nuevas visiones de las aplicaciones biomédicas de los nanomateriales fluorescentes.
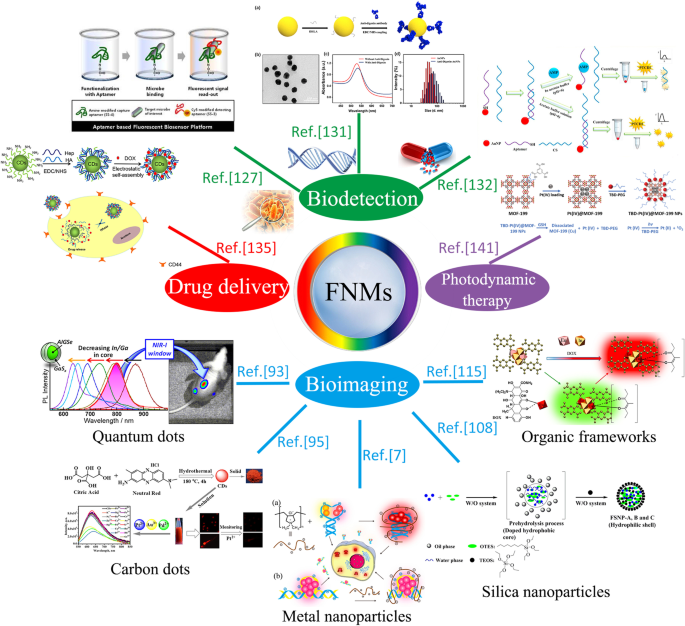
El diagrama general de las aplicaciones biomédicas de los nanomateriales fluorescentes
Considerando el gran potencial que poseen los nanomateriales fluorescentes en el área biomédica, esta revisión pone énfasis en los últimos avances y mejoras. Los científicos se han dedicado a la funcionalización de la superficie de nanomateriales fluorescentes y su desempeño en aplicaciones biomédicas. Sólo con estrategias de síntesis diseñadas razonablemente se pueden dotar a los materiales fluorescentes de la calidad de alta eficiencia PL y buena biocompatibilidad, que son vitales para sus aplicaciones en el campo biomédico. Esperamos que esta revisión sobre la síntesis y las aplicaciones de materiales fluorescentes pueda ser de alguna ayuda para que los lectores comprendan la tendencia general de desarrollo de los nanomateriales fluorescentes en la actualidad.
Síntesis de nanomateriales fluorescentes
Puntos cuánticos (cristales semiconductores)
Los puntos cuánticos (QD) fueron el punto de investigación en las últimas décadas debido a su amplia absorción y espectros de fotoluminiscencia simétricos, alto rendimiento cuántico, alta resistencia al fotoblanqueo, altos coeficientes de extinción molar y grandes cambios de Stokes efectivos [8]. En términos del mecanismo de formación de QD, cuando los portadores de carga (electrones y huecos) están restringidos por barreras potenciales a ciertas regiones, los semiconductores muestran efectos dramáticos de tamaño cuántico que resultan en el cambio del espectro de absorción y el espectro de fluorescencia. Las regiones pequeñas son menores que la longitud de onda de De Broglie de los portadores de carga o, de manera equivalente, el diámetro del nanocristal es menos del doble del radio de Bohr de los excitones en el material a granel [9]. Cuando los portadores de carga están confinados por barreras potenciales en tres dimensiones espaciales, se forman QD, que consisten principalmente en átomos de los grupos II-VI (CdSe, ZnS), III-V (GaAs, InP) o IV-VI (PbS, PbSe).
La síntesis de QD se informó por primera vez en 1982 [10, 11]. Los nanocristales y microcristales de semiconductores se cultivaron en matrices de vidrio. Con el desarrollo de materiales fluorescentes, los QD se han preparado mediante diferentes métodos, como el método de adsorción directa, el método de adsorción asistida por enlazador, los métodos in situ y la combinación de métodos de preparación anteriores. La combinación de métodos anteriores incluye la combinación de semiconductores preparados con precursores QD y la combinación de QD previamente preparados con precursores de semiconductores, en los que los semiconductores o QD se preparan por separado [12].
Después de una serie de investigaciones sobre la síntesis de QD, muchos investigadores informaron sobre el estudio de las propiedades fluorescentes de las QD. Bawendi y col. sintetizó las QD con distribuciones de tamaño estrechas mediante la introducción de precursores de semiconductores como el sulfuro de cadmio (CdS), el seleniuro de cadmio (CdSe) o el telururo de cadmio (CdTe) para investigar las propiedades ópticas dependientes del tamaño de las QD [13]. Desde entonces, el CdSe se convirtió en la composición química más común de los QD, y se han utilizado una variedad de modificaciones superficiales [14, 15, 16] o capa protectora inorgánica [13, 17] para proporcionar estabilidad coloidal.
Puntos de carbono
Los puntos de carbono (CD) son nanomateriales emergentes de la familia de los nanocarbonos con tamaños inferiores a 10 nm, que se obtuvieron por primera vez en la purificación de nanotubos de carbono de pared simple (SWCNT) por electroforesis en 2004 [18]. Es de destacar que los CD sustituyen gradualmente a los puntos cuánticos semiconductores debido a su alta solubilidad en agua, baja citotoxicidad, alta fotoestabilidad, emisión multicolor dependiente de la excitación, flexibilidad preferible en la modificación de la superficie, excelente permeabilidad celular y mejor biocompatibilidad [19, 20]. Generalmente, los CD comprenden principalmente puntos cuánticos de carbono (CQD) y puntos cuánticos de grafeno (GQD). Una gran cantidad de métodos sintéticos para CD con tamaños ajustables se puede dividir en dos grupos principales:métodos químicos y métodos físicos [21].
Métodos químicos sintéticos
Los métodos de síntesis química son los más comúnmente usados en la preparación de puntos de carbono porque los CD resultantes poseen excelentes propiedades, tales como solubilidad en agua superior, inercia química, baja toxicidad, facilidad de funcionalización y resistencia al fotoblanqueo. En general, los métodos de síntesis química incluyen síntesis electroquímica [22, 23], oxidación ácida [24, 25], carbonización hidrotermal [26], tratamiento asistido por microondas / ultrasónico [27,28,29], métodos de química en solución [30], síntesis admitida [31], etc.
Entre los numerosos métodos sintéticos, la síntesis electroquímica se ha informado repetidamente durante las últimas décadas. El grupo de Zhao informó sobre un método novedoso para preparar CD con baja citotoxicidad mediante síntesis de electrooxidación, mediante el cual los CD se prepararon mediante la oxidación de un electrodo de columna de grafito contra un electrodo de calomelanos saturado con un contraelectrodo de alambre de Pt en NaH 2 PO 4 solución acuosa [22]. A continuación, se ultrafiltró el sobrenadante a través de dispositivos de filtrado centrífugo para obtener los CD con fluorescencia azul y amarilla, respectivamente. Otro enfoque electroquímico directo fue informado recientemente por Qu et al., Para los GQD con un tamaño uniforme de 3-5 nm por oxidación electroquímica de un electrodo de grafeno en una solución tampón de fosfato [23]. El color fotoluminiscente (PL) de estas partículas era verde.
Mao y col. completó la síntesis de oxidación por combustión de CD en 2007 mezclando hollín de vela con un oxidante, seguido de reflujo, centrifugación y diálisis para purificar los CD. Los espectros de fotoluminiscencia de CD preparados tienen una amplia gama de colores, con longitudes de onda de pico de emisión que van desde 415 (violeta) a 615 nm (rojo anaranjado). Luego, los CD obtenidos se sometieron adicionalmente a electroforesis en gel de poliacrilamida para separar los CD con diferente caracterización óptica. La oxidación ácida también se ha utilizado ampliamente para la preparación de nanomateriales estables como puntos de carbono. Después del tratamiento ácido de los nanotubos de carbono / grafito y el reflujo, los CD resultantes de 3-4 nm presentaron un líquido transparente de color canela que emitía una fluorescencia de color amarillo brillante bajo luz ultravioleta y eran bastante estables en solución salina. Esto hizo que los CD con fluorescencia de onda larga (amarillo / naranja / rojo) posean una mejor penetración. La solución de CD se puede conservar a temperatura ambiente durante mucho tiempo y no se forman precipitados que provoquen la pérdida de fluorescencia [25].
La síntesis por microondas / ultrasónica se ha convertido de manera gradual y principalmente en una tecnología sintética auxiliar en el proceso de síntesis [32]. Los CD fluorescentes, de 3-5 nm de diámetro, fueron sintetizados por el grupo de Xiao mediante un enfoque económico, rápido y ecológico asistido por microondas [33]. La característica más destacada de este enfoque de un solo paso fue que tanto la formación como la funcionalización de los CD se completaron simultáneamente a través de la pirólisis de microondas derivada de los líquidos iónicos por primera vez [34]. El proceso de reacción se llevó a cabo en un horno microondas utilizando líquidos iónicos baratos como fuente de carbono y la solución cambió de incolora a marrón oscuro a medida que avanzaba el tiempo de reacción [35]. Tang y col. utilizó un método ultrasónico basado en glucosa o carbón activo como fuente de carbón para sintetizar CDs solubles en agua monodispersos. Emitían una fluorescencia brillante y colorida [28]. Asimismo, Vanesa Romero et al. obtuvieron puntos de carbono (CD) codopados con nitrógeno (N) y azufre (S) altamente fluorescentes después de la oxidación fotoquímica de los carbohidratos en vegetales. El codopaje de N y S aumenta el número de sitios activos en la superficie de los CD, mejorando así su rendimiento de luminiscencia [36]. Los puntos cuánticos de carbono dopado con nitrógeno (NCQD), una sonda fluorescente, se aplicaron con éxito a la determinación de doxiciclina [37]. Pathak y col. prepararon puntos de carbono codopados con nitrógeno y azufre (NSCD), que se sintetizaron a partir de tampón de tiourea y tris-acetato-etilendiamina mediante el método hidrotermal de microondas. Las NSCD se utilizaron para obtener imágenes de diversas bacterias patógenas y células epiteliales bucales humanas debido a la fluorometría multicolor [38].
Teniendo en cuenta que la mayoría de los métodos sintéticos mencionados anteriormente necesitaban ácido fuerte, varios pasos experimentales complicados y modificaciones adicionales con otros compuestos para mejorar la solubilidad en agua de los CD y mejorar su propiedad de fotoluminiscencia, algunos equipos de investigación explotaron la carbonización hidrotermal de la fotoluminiscencia de carbohidratos como quitosano, glucosa, ácido cítrico, etc. para evitar los complejos y prolongados procesos de purificación y funcionalización [39]. Yang y col. describieron un método sintético de un solo paso para CD fluorescentes altamente funcionalizados con amino con un rendimiento cuántico (QY) de 7.8% por carbonización hidrotermal de quitosano a una temperatura suave. Este método no necesitaba ni un disolvente ácido fuerte ni un reactivo de pasivación de superficie. Además, los grupos funcionales de la superficie de las CD mejoraron su solubilidad en agua y redujeron su potencial biotoxicidad [26]. Los puntos de carbono multidopados (MCD), con emisión brillante y sintonizable en color, se sintetizaron mediante el método de un solo recipiente sin ninguna pasivación superficial adicional. Los MCD sintetizados se doparon con abundantes elementos biogénicos (O, N, P) y, por lo tanto, muestran una fuerte emisión fluorescente y una característica dependiente de la longitud de onda de excitación, buena solubilidad en agua, alta estabilidad óptica y estabilidad iónica. Los MCD no solo pueden detectar de forma selectiva y sensible Fe 3+ bajo luz azul de detección a 15.9 nm, pero también mida el Fe intracelular 3+ a través de imágenes de fluorescencia multicolor [40].
Para los métodos de química en solución, la condensación oxidativa de grupos arilo se ha aplicado con éxito a la preparación de GQD durante las últimas décadas. El grupo de Li produjo GQD coloidales estables con los tamaños y estructuras deseados con una estrategia de solubilización. Este método logró la capacidad de ajuste de tamaño y una distribución de tamaño estrecha de los CD sin ningún proceso de separación de tamaño impráctico [30]. En lo que respecta al procedimiento sintético admitido, varios equipos de investigación lo han aprovechado para completar la síntesis de nanomateriales monodispersos, como los CD nanométricos. El grupo de Zhu adoptó esferas de sílice mesoporosa (MS) como nanoreactores y ácido cítrico como precursor de carbono y se prepararon CD hidrófilos con tamaños de 1,5 a 2,5 nm mediante un método de impregnación. Los CD con una alta eficiencia de fotoluminiscencia del 23% fueron capaces de emitir una fuerte luminiscencia azul y presentar excelentes propiedades de conversión de luminiscencia [31]. Yan et al. mediante el método solvotermal, utilizando ácido cítrico anhidro como fuente de carbono y 2,3-fenazindiamina como fuente de nitrógeno. Los Y-CD con abundantes grupos carboxilo mostraron un rendimiento cuántico de fluorescencia respetable (24%), desplazamiento de Stokes de 188 nm, alta sensibilidad y excelente estabilidad [41]. Los métodos sintéticos y las propiedades de los CD se presentan en la Tabla 1.
Métodos físicos sintéticos
En general, los métodos sintéticos físicos incluyen principalmente la descarga de arco, la ablación / pasivación con láser y el tratamiento con plasma. Xu y sus compañeros de trabajo oxidaron el hollín de la descarga de arco con HNO 3 y luego se separó la suspensión mediante electroforesis en gel en SWCNT. Finalmente aislaron la banda de rápido movimiento de nanopartículas de puntos de carbono altamente fluorescentes [18]. Li et al. mediante un abordaje de ablación con láser suave [44]. Además, Gokus y sus colaboradores demostraron que el uso de plasma de oxígeno podría inducir una fuerte fluorescencia en grafeno de capa única [45].
Nanopartículas de carbono
Las nanopartículas de carbono fluorescente, con su citotoxicidad reducida, su resistencia al fotoblanqueo y su mayor biocompatibilidad, están atrayendo cada vez más la atención de las bioimágenes y otras aplicaciones biomédicas. En comparación con los tamaños típicos de los puntos de carbono dentro de 1 a 6 nm, los tamaños de las nanopartículas de carbono son más de 20 nm, lo que evita la molestia de separar, purificar y recolectar [46]. Los métodos de síntesis de nanopartículas de carbono son similares a los puntos de carbono, incluida la carbonización hidrotermal, el tratamiento con microondas, el método de ablación química y la ablación con láser. Estos métodos tienen sus propias ventajas, pero no pueden controlar eficazmente el tamaño de las nanopartículas. La carbonización electroquímica es un método de un solo paso que puede controlar el tamaño y las propiedades de luminiscencia de las nanopartículas de carbono. Desafortunadamente, hay muy pocos sustratos disponibles para este método. En la actualidad, se han informado algunos métodos nuevos e intrigantes, como el método de combustión del pentóxido de fósforo [47].
En los últimos años, las nanopartículas de carbono adecuadas para aplicaciones biomédicas se sintetizan con métodos modificados. Santu y col. resolvió la síntesis de nanopartículas de carbono rojo fluorescente de alta calidad mediante carbonización controlada de resorcinol [48]. Este enfoque implica el acoplamiento de fenol oxidativo asociado con la deshidratación para formar nanopartículas de carbono fluorescentes rojas. Anara y col. nanopartículas de carbono fluorescentes sintetizadas con un rendimiento cuántico del 6,08% utilizando un método hidrotermal modificado. En comparación con los métodos convencionales que requieren un tratamiento térmico prolongado de hasta varias horas, este método acortó el tiempo de reacción a menos de 30 min, logrando la síntesis rápida de nanopartículas de carbono fluorescentes [46].
Nanotubos de carbono
Los nanotubos de carbono unidimensionales (1D) han generado una enorme atención en el campo biomédico en virtud de sus excelentes propiedades electrónicas y ópticas. Los nanotubos de carbono se pueden dividir en nanotubos de carbono de pared simple (SWCNT) y nanotubos de carbono de paredes múltiples (MWCNT) según el número de capas de grafeno cilíndricas. Mientras que los SWCNT se componen de una sola capa de lámina de grafeno enrollada en un cilindro, los MWCNT comprenden varias capas concéntricas de lámina de grafeno. El diámetro exterior de los nanotubos de carbono es inferior a 100 nm, pero sus longitudes pueden alcanzar hasta varios milímetros, lo que da lugar a una relación de aspecto muy alta y una gran superficie [49]. Además, la disposición única de los átomos de carbono en los nanotubos de carbono forma una rica conjugación de electrones π fuera del nanotubo [50]. Además, los nanotubos de carbono están dotados de una fuerte absorción y fluorescencia en la región NIR [51]. Todas estas características contribuyen a la interacción efectiva con biomoléculas, lo que hace que los nanotubos de carbono sean un candidato ideal para aplicaciones biomédicas.
Los métodos sintéticos tienen una gran influencia en el diámetro, la longitud, la estructura, la quiralidad y la calidad de los nanotubos de carbono y, mientras tanto, se debe considerar si este método es adecuado para la producción a gran escala. Los métodos comúnmente utilizados incluyen la descarga por arco [52], la ablación con láser [53] y la deposición química en fase de vapor [54]. Además de esto, los nanotubos de carbono deben funcionalizarse para mejorar su solubilidad y evitar que se agreguen en solventes y medios biológicos. La funcionalización covalente introduciría defectos en la estructura de los nanotubos de carbono, lo que provocaría una disminución drástica o incluso una pérdida completa de su fluorescencia NIR. La funcionalización no covalente con moléculas anfifílicas como los polímeros preservaría la estructura y las propiedades fluorescentes de los nanotubos de carbono, pero reduciría el QY de los nanotubos de carbono. Para superar estos obstáculos, se han informado recientemente métodos novedosos para sintetizar y funcionalizar nanotubos de carbono. Lee y col. informaron de que la adición de ditiotreitol, que es un agente reductor, puede mejorar la QY fluorescente de los SWCNT por primera vez, lo que da como resultado fluoróforos con un brillo equivalente al de los QD [55]. Hou y col. investigó la adición de ditiotreitol a SWCNT funcionalizados con una variedad de tensioactivos. Para los SWCNT envueltos en ADN y SDS, la QY fluorescente de ellos aumentó significativamente, mientras que se observó extinción de la fluorescencia en diferentes grados para otros tensioactivos [56]. Como resultado, la adición de ditiotreitol al ADN o los SWCNT envueltos en SDS son soluciones factibles para lograr la aplicación de nanotubos de carbono en biomedicina.
Nanomateriales basados en grafeno
Como nanomateriales de carbono bidimensionales, el grafeno y sus derivados se han explorado ampliamente para una variedad de aplicaciones biomédicas, como la bioimagen y la administración de fármacos. Los nanomateriales de grafeno incluyen nanohojas de grafeno, nanoplacas de óxido de grafeno (GO) y óxido de grafeno reducido (rGO). Tienen áreas de superficie elevadas y propiedades de superficie únicas que permiten interacciones no covalentes con moléculas de colorante, biomoléculas y fármacos insolubles en agua. Muchos investigadores han informado sobre diferentes métodos de preparación de grafeno desde que se preparó con éxito por primera vez en 2004. Los métodos sintéticos de nanomateriales de grafeno se pueden clasificar en dos categorías, de arriba hacia abajo y de abajo hacia arriba.
Los métodos de arriba hacia abajo implican el aislamiento de capas de grafito apiladas para formar láminas de grafeno, incluida la exfoliación mecánica [57], la exfoliación a base de solventes [58] y la exfoliación electroquímica [59]. Gu y col. Estudió sistemáticamente la exfoliación a base de solventes asistida por ultrasonido y descubrió que las ondas ultrasónicas tienen un buen efecto de exfoliación. También pueden afectar la distribución del tamaño y el grosor de las láminas de grafeno, lo que hace posible una síntesis controlable. Los enfoques de abajo hacia arriba implican la reorganización de los átomos de carbono utilizando fuentes de carbono alternativas. El crecimiento epitaxial [60] y la deposición química en fase de vapor (CVD) [61] son los métodos de síntesis ascendente más comúnmente utilizados. GO hojas compuestas por muchos sp 2 Los dominios aislados por grupos que contienen oxígeno se pueden sintetizar utilizando el método de Hummer. Variaciones en los tamaños de estos sp 2 Los dominios hacen que el PL de las hojas GO varíe ampliamente entre 500 y 800 nm [62]. rGO se deriva de GO mediante reducción química utilizando agentes reductores como hidroquinona e hidracina. En comparación con GO, la fluorescencia de rGO mostró una emisión desplazada al azul en la región UV junto con la extinción de la fluorescencia, que se atribuye a las vías de percolación entre la sp 2 cristalina recién formada racimos [63]. Akbari y col. aclaró que la proporción de sp 3 / sp 2 dominios en hojas GO determina sus espectros de fluorescencia. Por lo tanto, GO es un nanomaterial fluorescente prometedor en una amplia gama de longitudes de onda con diferentes grados de reducción, que se puede utilizar en aplicaciones biomédicas.
Nanomateriales metálicos
Los átomos de metales nobles presentan menos citotoxicidad en comparación con los QD al mismo tiempo. Las nanopartículas de oro, plata y cobre han recibido una atención cada vez mayor y se aplican a una gran cantidad de campos. En los campos biomédicos, los efectos de la mecánica cuántica de las nanopartículas de oro, como la emisión de fotoluminiscencia o la resonancia de plasmón, hacen que las nanopartículas de oro (AuNP) sean un candidato ideal para otro nanosensor in vivo con baja citotoxicidad [64, 65].
Los AuNP han atraído un gran interés científico en virtud de su facilidad de síntesis y propiedades únicas, y se han informado diversos métodos sintéticos. Como uno de los métodos más importantes, los métodos químicos se realizan generalmente tratando una solución acuosa de cloroaurato con agentes reductores en presencia de un agente estabilizante. El ácido cítrico es el más utilizado, que puede actuar tanto como estabilizador como como agente reductor [66]. Sin embargo, los AuNP estabilizados con ácido cítrico pueden sufrir una acumulación irreversible durante el desarrollo de la funcionalización con ligandos de tiolato. Este problema puede superarse haciendo que la reacción tenga lugar en presencia de polímeros solubles en agua, tensioactivos o agentes de protección que ayudan a proporcionar una mayor estabilidad y previenen la agregación de nanopartículas. El tamaño y la forma de los AuNP se pueden controlar cambiando la proporción de citrato de oro, los agentes modificadores de la superficie o las condiciones de reacción. Con el método de emulsificación ultrasónica en un recipiente, Zhang y sus compañeros de trabajo cargaron conjuntamente Bis (4- (N- (2-naftil) fenilamino) fenil) -fumaronitrilo y AuNPs en micelas para obtener la nanoonda [67]. Sobre todo, la nanosensda obtenida, con gran potencial para ser aplicada en diagnóstico por imagen y diagnóstico in vivo de tumores, procesó una excelente capacidad de obtención de imágenes de fluorescencia, a pesar de la existencia de nanopartículas de oro. Aunque los AuNP no son tóxicos en determinadas condiciones experimentales, es necesario examinar a fondo la toxicidad y los efectos secundarios [68].
Se ha prestado mucha atención a los nanoclusores de Ag fluorescente debido a sus propiedades físicas y químicas únicas. El proceso de síntesis de tales nanoclusters se clasifica mediante el andamio estabilizador en oligonucleótidos de ADN, péptidos, proteínas, dendrímeros y polímeros. Además, una extensa literatura ha demostrado alguna síntesis verde, como la aplicación de extractos de tallo acuosos de D. trifoliata y S. alba para optimizar las condiciones de preparación [69].
Los nanoclusters de Cu (Cu NC) se utilizan relativamente ampliamente como materiales de metales nobles, pero su síntesis aún es escasa debido a su vulnerabilidad a la oxidación. Recientemente, Kawasaki et al. prepararon con éxito Cu NC estables mediante un método de poliol asistido por microondas [70]. El ADN podría usarse como molde para la síntesis de Cu NC fluorescentes. Mohir y col. propuso un método basado en ADN bicatenario en solución para obtener Cu NC con alta selectividad [71]. Utilizando las propiedades fluorescentes de Cu NC, se aprovechó con éxito como un indicador de señal de encendido fluorescente eficaz para la determinación selectiva de RDX [72].
Nanopartículas de sílice
Teniendo en cuenta las propiedades de transparencia, estabilidad mecánica, robustez y estabilización de los fluoróforos incrustados, las nanopartículas de sílice se aplican ampliamente en dominios biológicos. Por ejemplo, NP de núcleo / capa de sílice aplicadas para la detección intracelular de Zn 2+ y H 2 PO 4 - en células vivas se sintetizaron como nanosensores fluorescentes "apagado / encendido". En los últimos años, se han sintetizado nanopartículas de sílice dopadas con tintes orgánicos y se han utilizado ampliamente en muchas aplicaciones, como la biodetección [73]. Los métodos de síntesis más utilizados para nanopartículas de sílice son el método Stöber y el método de microemulsión inversa. El método Stöber, descrito por primera vez en la década de 1960 [74], implica la hidrólisis de silicatos de alquilo y la posterior condensación de ácido silícico en soluciones alcohólicas catalizadas por la adición de amoníaco. El segundo método de formación de NP de sílice, el método de microemulsión inversa, implica la reacción de silicatos de alquilo, típicamente TEOS, dentro de las gotas de agua de una microemulsión de agua en aceite [75]. He et al. preparó tres tipos de nanopartículas de sílice dopantes con colorante con el método Stöber y el método de microemulsión inversa incorporando colorante en el núcleo de la partícula. Algunas moléculas responden funcionalmente a Zn 2+ se depositan sobre la superficie de las partículas [76]. Las nanopartículas de sílice fluorescente se utilizaron para imágenes fluorescentes de Zn intracelular 2+ (H 2 PO 4 - ) en células HeLa. Cuando Zn 2+ se agregó al Zn 2+ proporcional nanosensor, las nanopartículas mostraron la capacidad de detectar ratiométricamente la concentración de H 2 PO 4 - .
En general, los nanocompuestos de sílice con buena monodispersidad y biocompatibilidad pueden modificarse fácilmente con grupos funcionales [77,78,79]. Lee y col. nanopartículas magnéticas dopadas y tintes fluorescentes en nanopartículas de sílice. Estos nanocompuestos de sílice se pueden utilizar no solo como sondas de formación de imágenes multimodales para la resonancia magnética (RM) y la formación de imágenes de fluorescencia, sino también como portadores de administración de fármacos contra el cáncer [80]. En pocas palabras, las partículas de sílice podrían ser un lugar de investigación en tales casos con usos muy extendidos.
Fósforos
Los fósforos se utilizan ampliamente en biomedicina debido a sus ventajas únicas para reducir la autofluorescencia y la interferencia de dispersión de luz de los tejidos. En general, los fósforos se componen de materiales huésped e iones dopados [81]. Entre los materiales hospedantes de fósforos, el óxido de itrio (Y 2 O 3 ) es más que prometedor debido a su baja fotodurabilidad y su energía fonética. Los lantánidos están en gran parte dopados en fósforos considerando sus abundantes niveles de electrones y canales de transferencia de energía. Se han informado numerosos métodos para la preparación de fósforos, incluidos los procesos hidrotermal [82], pirólisis por aspersión de llama [83], sol-gel [84] y coprecipitación [85].
La síntesis hidrotermal se perfila como un proceso ideal, que ha demostrado ser eficiente y económico para la síntesis de fósforos. Yu y col. sintetizado Y 2 O 3 :Eu 3+ fósforos por método hidrotermal en presencia de citrato de sodio [82]. El concentrado de citrato de sodio, la cantidad de adición de NaOH y Eu en el proceso hidrotermal decidieron las propiedades de los fósforos obtenidos. La pirólisis por aspersión de llama es un método prometedor para la síntesis rápida y consecutiva de fósforos a base de óxidos. En comparación con los métodos convencionales, este método proporciona fósforos con alta cristalinidad y distribución homogénea de dopantes. Khan y col. producido con éxito Tb 3+ –Doped Y 2 O 3 fósforos que tenían aproximadamente 100 nm de diámetro con una distribución de tamaño estrecha utilizando pirólisis por aspersión de llama [83]. En su método, la sal alcalina se mezcló con otros precursores de nitrato metálico, que controlaron eficazmente la distribución del tamaño en un rango estrecho. The sol–gel synthesis route offers several advantages, such as high homogeneity and purity, reduced synthesis time, uniform particle morphology, and narrow particle size distribution [86]. Leonardo et al. obtained Sm 3+ doped SiO2 -Gd2 O3 phosphors by a sol–gel process [84]. Co-precipitation is a common and simple method for synthesizing crystalline phosphors, which ensures high homogeneity and controlled morphology characteristics. Perhaita et al. reported that the phase composition of the phosphors strongly depends on the pH during the precipitation [85].
Organic Frameworks
Covalent-organic frameworks (COFs) are new porous crystalline materials possessing outstanding stability, adsorption and low toxicity. The design of fluorescent small organic molecules with a combination of fluorescence determination methods can be used to construct more efficient nanoprobes [87]. For selective 2,4,6-trinitrophenol (TNP) determination, a novel Naphthalimide-Benzothiazole conjugate was prepared as colorimetric and fluorescent nanoprobe. The fluorescence emission peaks of receptor were selectively quenched by TNP with a limit of detection as low as 1.613 × 10 –10 M.
Metal organic frameworks (MOFs) are a kind of new generation multifunctional inorganic–organic materials with various holes and functionalized 3D crystalline structures formed by metal ions and linkers. MOFs show potential applications in separation, catalysis and other aspects due to unique attributes such as excellent chemical tenability, specific surface area and confinement of the pores. Some of the MOFs are luminescent and the quantum yield as well as light intensity will be influenced by temperature and excitation wavelength [88]. With the addition of doxycycline, Yu et al. synthesized a new functional metal–organic framework of pyromellitic acid and europium, which exhibited remarkable fluorescence enhancement at 526 nm and 617 nm. Results showed that both fluorescence intensities were positively correlated with the doxycycline concentration. The unique fluorescence response of the system could discriminate doxycycline from other tetracycline antibiotics with high selectivity.
Biomedical Applications of Fluorescent Nanomaterials
Bioimaging
Quantum Dots for Bioimaging
Fluorescent nanomaterials have been widely used in bioimaging. Compared with conventional organic fluorescent molecules, fluorescent nanomaterials are equipped with many superior properties such as high photostability, tunable emission spectra and high quantum yields [89].
As early as 1998, QDs were first successfully applied in biological imaging [90]. Since then, applications of QDs in this field have been springing up gradually. Chen’s group applied it in bioimaging and nuclear targeting with great stability and biocompatibility in living cells [91]. In spite of the extremely high sensitivity and spatial resolution of QDs, poor performances on hydrophilicity and biocompatibility hindered their applications in bioimaging in vivo. To tackle this problem, it has been found that the water-solubility of QDs can be greatly improved by attaching thiol or other hydrophilic groups to the surface of quantum dots [92]. In the same way, with the intention of improving the effectiveness and specificity of in vivo targeted imaging, targeting molecules are attached to the surface of QDs. Furthermore, the wavelength region of the emission light can be controlled by altering the size of QDs.
The combination of QDs and inorganic metal ions can optimize the application of QDs in bioimaging because the QDs’ defect-site PL peaks will be utterly removed by controlling the proportion of doped inorganic metal ions. Kuwabata, S et al. modulated the degree of Ga 3+ doping in Ag–In–Se QDs. Thus, the QDs’ defect-site PL peaks were completely removed and a sharp band-edge emission peak come into appearance [93]. They found a blue shift of the band-edge PL peak ranging from 890 to 630 nm which could be credited to the fact that the energy gap of QDs was enlarged by Ga 3+ doping. After injecting a mouse with QDs, the potential of AIGSe@GaSx core–shell QDs for bioimaging turned out satisfying. The imaging effect of this kind of QDs in mice is demonstrated in Fig. 2. However, sensing of mid-IR wavelengths is challenging due to increased dark currents and noise. HgTe QDs synthesized by colloidal method is a promising candidate for IR bioimaging by virtue of lower dark currents, higher-temperature operation, and higher detectivity [94].
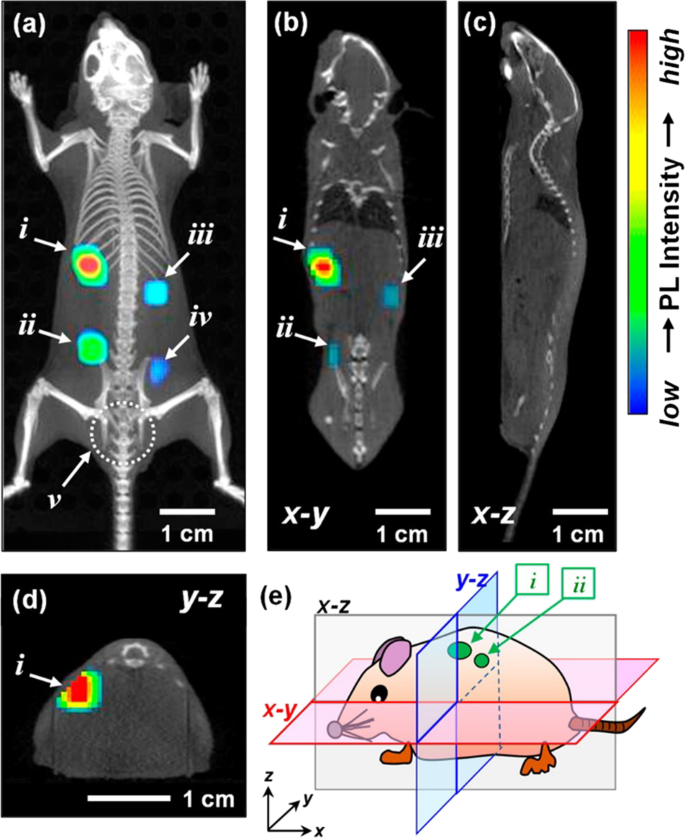
Three-dimensional PL image superimposed on an X-ray CT image of the mouse subcutaneously injected with DSPC-AIGSe@GaSx liposome dispersions (each 50 mm 3 ) in the back [78]
Carbon Dots for Bioimaging
The poor photostability of current fluorescent nanomaterials hinders their long-term bioimaging to a large extent. To overcome this limitation, CDs have been studied for bioimaging and some positive results have been obtained due to the great performance on PL efficiency. Enormous efforts have been put to improve their water solubility and lower their toxicity in organisms. At present, most CDs are facing a barrier in bioimaging, that is, their short-wavelength excitation disables deep penetration in tissue. Aside from this, being exposed under the short-wavelength for a long time could do irreversible damages to living cells and tissues. As shown in Fig. 3, with the purpose of overcoming this deficiency, Gao et al. designed fluorescent CDs with red emission which were successfully used for bioimaging of noble metal ions (Pt 2+ , Au 3+ , Pd 2+ ) in cells and zebrafish [95]. Sun and co-workers first studied the near infrared (NIR) imaging of CDs in vivo using mice as a model. Recently, it was reported that molecules or polymers containing plentiful sulfoxide or carbonyl groups can enhance NIR fluorescence through the surface modification. As shown in Fig. 4, under NIR excitation, sulfoxide or carbonyl groups are bound to the outer layers and the edges of the CDs. Thus, electron transitions are promoted, influencing the optical bandgap [96].
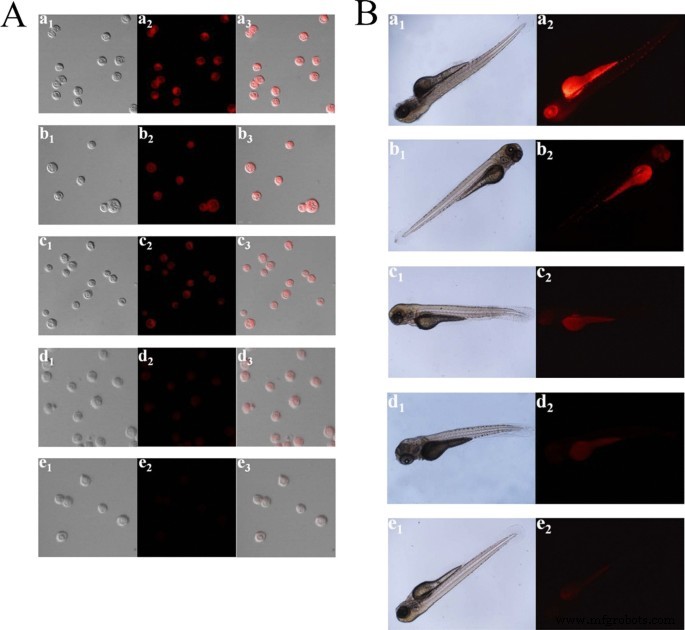
A Confocal imaging of Pt 2+ in PC12 cells. (a1–e1) Bright field images. (a2–e2) Black field images of the CDs in PC12 cells with the different concentrations of Pt 2+ (0, 25, 50, 150, and 300 μM). (a3–e3) Overlay images. B Fluorescence imaging of Pt 2+ in ZF. (a1–e1) Bright field images. (a2–e2) Fluorescence images of the CDs in ZF with the various concentrations of Pt 2+ (0, 30, 60, 100, and 150 μM) [80]
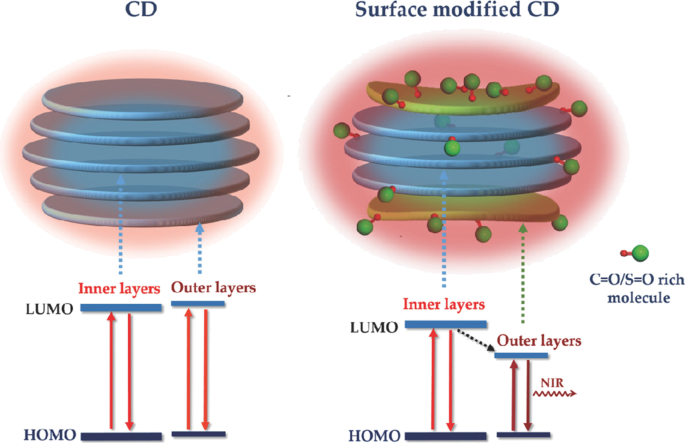
Schematic of structure and energy level alignments of nontreated CDs (left column) and CDs modified with S = O/C = O‐rich molecules (right column). The red (oxygen atom) and green double‐bonded balls represent the C = O/S = O‐rich molecule [81]
Carbon Nanoparticles for Bioimaging
In the field of bioimaging, fluorescent carbon nanoparticles show unique chemical and optical properties over traditional fluorescence probes. Different size, shape and elemental composition make carbon nanoparticles with different features. The biomedical fields are always seeking the most promising fluorescent carbon nanoparticles. Gaurav et al. obtained both larger and smaller size carbon nanoparticles with laser ablation method [97]. Both green and blue fluorescence were observed in the cells incubated with the carbon nanoparticles, suggesting their different sizes. Cell viability results indicated that the prepared carbon nanoparticles were nontoxic and safe for bioimaging applications. Shazid et al. employed carbonization method to obtain fluorescenct carbon nanoparticles derived from biocompatible hyaluronic acid. Both the in vitro and in vivo bioimaging studies showed that the prepared carbon nanoparticles would be reliable and stable for opticle imaging. Moreover, based on the experimenal data, their cytotoxicity was proved to be tolerable for biomedical applications.
Carbon Nanotubes for Bioimaging
Fluorescence of carbon nanotubes in the NIR is attracting high attention for their good light penetration depth in biological tissues. However, their low quantum yield requires for considerable excitation doses, leading to a fair degree of blue-shift and failure of penetrating live tissue. Mandal et al. reported that bright and biocompatible p-nitroaryl functionalized SWCNTs, encapsulated in phospholipid-polyethylene glycol, are suitable for bioimaging applications. The prepared SWCNTs enabled high signal-to-noise ratio imaging in live brain tissues using ultra-low excitation intensities. Their 1160 nm emissions in the NIR guarantee that they will provide optimal fluorescence imaging results [98]. Ceppi et al. applied SWCNT-based fluorescence imaging to debulking surgery in an ovarian cancer mouse model. SWCNTs are coupled to an M13 bacteriophage carrying modified peptide binding to the SPARC protein, which is overexpressed in ovarian cancer, leading to real-time imaging to guide intraoperative tumor debulking. This imaging system enables detection in the NIR window with a pixel-limited resolution of 200 μm, demonstrating real potential in fluorescence imaging guided surguries for patients [99].
Furthermore, fluorescent moieties can be conjugated by a carbon nanotube backbone, which integrate strong fluorescent ability with robust mechanism strength, exhibiting ideal bioimgaing results. Katharina et al. functionalized SWCNTs with an amphiphilic C18 -alkylated polymer conjugated with bright perylene bisimide fluorophores. The polymers wrapping around the SWCNT backbones not only increase their water dispersibility but also promote their biocompatibility by providing a shield. In vitro studies on HeLa cells demonstrated that the biocompatibility of SWCNTs is dramatically improved. In microscopy studies, direct imaging of the SWCNTs' cellular uptake via perylene bisimide and SWCNT emission proved their potential for bioimging [100]. Park et al. combined carbon nanotubes with mussel adhesive proteins which can be specifically targeted at tumors in tissue. They then made carbon nanotubes conjugated with a ZW800 NIR fluorophore to obtain NIR fluorescence imaging [101]. The prepared carbon nanotube probes react with a specific tumor in one hour and can be easily eliminated via urine, demonstrating great value as tumor imaging and detecting agent.
Graphene-Based Nanomaterials for Bioimaging
Large surface area and feasible further functionalization make graphene-based nanomaterials a promising candidate for biomedical applications. However, as a result of their chemical sturctures, graphene nanosheets lack photoluminescence and rGO only display weak fluorescence, which makes it difficult to be utilized in bioimgaing applications. Many researchers attempted to resolve this problem by conjugation of fluorescent dyes and probes onto the large surface of graphene and its derivatives. Sun y col. reported an assembly strategy to prepare fluorescence probe RACD functionalized a single layer GO via π-π interaction and hydrogen bonding. The resluting nanomaterials exhibited that the fluorescent probes reduce the aggregation degree and acquire very well monodispersion, hydrophilicity and photostability, which is attributed to the strong synergy between RACD and GO [102]. Even so, fluorescence quenching remains a critical issue for these materials. In addition, the biocompatibility and toxicity of polymers applied to connect graphene-based materials and fluorescent moieties have not been adequately investigated. These facts appeal for alternative solutions to utilize graphene and its derivatives in bioimaging applications. Georgia et al. developed intrinsically photoluminescent graphene derivatives that show desirable biocompatibility and tunable fluorescence properties [103]. They can be organophilic or hydrophilic with different amine functionalization dodecylamine and hexamethylenediamine, respectively. The intrinsic fluorescent graphene-based nanomaterials possess great potential in a variety fileds of bioimgaing.
Metal Nanomaterials for Bioimaging
In recent years, fluorescent metal nanoparticles have shown great potential in bioimaging for improved disease diagnosis and treatment [104]. Gold is the most commonly used metal for bioimaging. The surface of AuNPs can be easily modified with various biomolecules such as peptides, proteins, antibodies, enzymes, and nucleic acids. These biomolecules can interact with specific cells or organelles in vivo, which makes it possible for AuNPs to be used for targeted optical imaging. Gao et al. reported a real-time in situ imaging of nucleus by AuNPs fabricated with bifunctional peptides constructed with both Au-binding affinity and nucleus-targeting ability. The bifunctional peptides showed strong binding affinity toward AuNPs and ensured good surface coverage of the nanoparticles, which made it stable and efficient for precise bioimaging of the nucleus in cells [105]. The Au-Se bond is considered as a better candidate than the Au–S bond to link the peptides and AuNPs due to the stronger ability against interference of intracellular thiol. Pan et al. prepared the Au-Se-peptide nanoprobes through a direct freezing process. The obtained nanoprobe was successfully applied to identify autophagy and apoptosis in chemotherapeutic drug treated cancer cells [106].
As a novel fluorescent imaging technology, DNA-templated silver nanoclusters (DNA-Ag NCs) have aroused the attention of many scientists due to their unique properties, especially the tunable fluorescence emission range relying on DNA sequences. However, the highly negatively charged DNA backbones have always been a great obstacle for the expansive applications in bioimaging because of poor stability as well as poor cell permeability in physiological environment. It is also noteworthy that the PL property and fluorescent efficiency of DNA-Ag NCs are far from satisfying. As a result, figuring out how to neutralize the negative charge on the surface of DNA strands is of great urgency for researchers. Recently, Lyu and co-workers successfully modified fluorescent DNA-Ag NCs with cationic polyelectrolytes via electrostatic force between the positively charged polyelectrolytes and the negatively charged phosphate groups of the DNA strands, leading to a threefold fluorescence intensity enhancement [7] (Fig. 5). Li et al. reported a facile strategy to make gold nanoclusters with positive charge and silver nanoclusters with negative charge form aggregates by electrostatic interactions. An incredible 40-fold fluorescence intensity enhancement was obtained. Results demonstrated that the physiological stability improved a lot and the cell permeability was also enhanced, which promises its practical applications in the future.
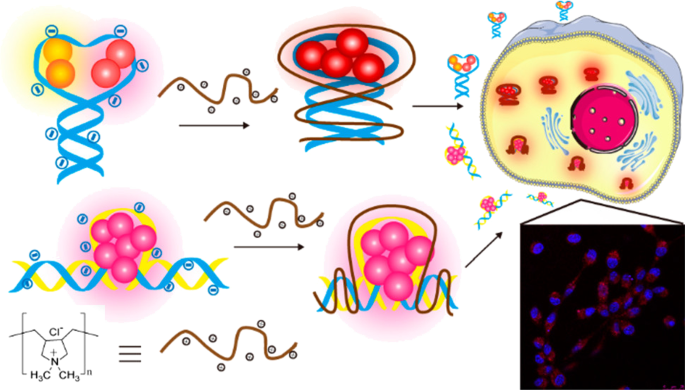
Formation of FL DNA–Ag NC–Cationic Polyelectrolyte Complexes for Cell Imaging [7]
Silica Nanoparticles for Bioimaging
Dye-doped fluorescent silica nanoparticles emerge with great potential for bioimaging as a novel and ideal platform for the monitoring of living cells and the whole body. The outer silica shell matrix protects fluorophores from outside chemical reaction factors as well as provides a hydrophilic shell for the inside insoluble nanoparticles, which renders the enhanced photo-stability and biocompatibility to the organic fluorescent dyes. Benefiting from the robust structure of silica matrices, dye-doped fluorescent silica nanoparticles have been presented with several superior properties including good biocompatibility, hydrophilic features, and high fluorescence intensity [107].
Jiao et al. also constructed a local hydrophobic cage in dye-doped fluorescent silica nanoparticles to improve their optical properties, which solves the problems of aggregation-caused quenching (ACQ) and poor photostability in aqueous media by organic fluorescence dyes benefiting from the robust structure of silica nanoparticles [108]. In addition, compared with free dyes, the fluorescent intensity both in water solution and living cells demonstrated a 12.3-fold enhancement due to the limitation of molecular motion, indicating a significant development for silica nanoparticles in biomedical applications. QDs have been developed for bioimaging both in vivo and vitro owing to their excellent optical qualities. However, a critical obstacle faced in QDs’ application in vivo is their poor biocompatibility. Inspired by the organic dye-conjugated silica-NPs, QDs-embedded silica-NPs have also been invented with the advantage that the excellent optical qualities of QDs can be retained, while the silica-NPs coat improves their biocompatibility to a large extent simultaneously. Darwish et al. reported that many QDs could be assembled around a central silica nanoparticle to form supra-NP assemblies. It was expected to be used for enhanced bioimaging because of their higher sensitivity and superior signal-to-background ratios [109]. There is reason to believe that silica-NPs conjugated with fluorescent nanomaterials with ideal optical properties will still be the dominant research interest in the future.
Phosphors for Bioimaging
For bioimaging, the sizes of phosphors need to be controlled so that they are small enough to be integrated with living cells. Furthermore, the aggregation of particles should be avoided for biocompatibility. Hence, the control of both particle sizes and dispersity in an aqueous solution is essential for the bioimaging application of the phosphors. Atabaev et al. prepared Eu, Gd-codoped Y2 O3 phosphors which had a spherical morphology within the range 61–69 nm. Enhanced PL emission and low toxicity made these phosphors suitable for bioimgaing applications [110].
Upconversion nanomaterials are able to convert lower-energy near-infrared photons to higher-energy ones as emission. This anti-Stokes photoluminescence process will lead to low background noise, large tissue penetration depth, and low photo-damage in bioimaging applications [111]. Lanthanide-based phosphors are able to show upconversion emission owing to their photodurability and low phonon energy. Nallusamy et al. reported a NIR–NIR bioimaging system based on Er 3+ :Y2 O3 phosphors by using NIR emission at 1550 nm under 980 nm excitation, which can allow a deeper penetration depth into biological tissues than ultraviolet or visible light excitation [112]. In addition, the surface of Er 3+ :Y2 O3 was electrostatically PEGylated to improve the chemical durability and dispersion stability under physiological conditions. Thakur et al. synthesized Ho 3+ /Yb 3+ co-doped GdVO4 phosphors via a modified sol–gel method. The prepared phosphors showed brilliant red upconversion emission under NIR excitation, which may be useful in bioimaging of the biomolecules [113].
Organic Frameworks for Bioimaging
Careful selection of MOF constituents can yield crystals of ultrahigh porosity and high thermal and chemical stability, with some of them being luminescent [114]. Recently, Sava Gallis’s group described a novel multifunctional MOF material platform which showed a wide spectral region from 614 to 1350 nm covering the deep red to NIR region. Both porosity and tunable emission properties made them highly suitable for in vivo bioimaging [115]. What’s more, to overcome the obstacle of MOF’s low selectivity towards malignant tissues, Liu et al. developed a target-induced bioimaging by conjugating DNA aptamers using ZrMOF nanoparticles as quenchers [116]. Based on the quenching of ZrMOF nanoparticles, target-induced bioimaging is achieved upon binding with the target.
Biodetection
Since fluorescent nanomaterials can amplify the fluorescent signals significantly and be compatible with organisms, there is increasingly more research on their application in the rapid detection of biomolecules [117]. It will shorten the analysis time to a large extent if we are able to establish a real-time detection system by fluorescent nanomaterials. It has been discovered that multiple detection can be achieved by using QDs probes simultaneously [118, 119].
Pathogen Detection
Pathogens have been an unignorable threat to human health for centuries and these include many types of microorganisms ranging from bacteria (pathogenic Escherichia coli, Salmonella, and Streptococcus pneumoniae ) and viruses (Coronavirus, Influenza virus and hepatitis virus ). However, conventional methods for pathogen detection still need improvement of detection limits and detection speeds. For their applications in detecting pathogens, Tan and co-workers reported a bioconjugated nanoparticle-based biodetection for in situ pathogen quantification, which cost less than 20 min [120]. Tan’s success promises that quick and convenient pathogen detection is possible and can be achieved with these ingenious nanomaterials in the future. Here, we list diverse pathogens detected by fluorescent nanomaterials as shown in Table 2 [119, 121,122,123,124,125,126,127,128,129].
Nucleic Acid Detection
Apart from pathogen detection, fluorescent nanomaterials have also aroused more and more interest of scientists in the detection of DNA. Owing to the merits that a number of biomolecules can be attached to the surface of fluorescent nanomaterials, the signal intensity of fluorescent nanomaterials in DNA detection can be enhanced significantly. Tan and co-workers developed an DNA detection method to detect gene products using bioconjugated dye-doped fluorescent silica nanoparticle with high sensitivity and photostability [130]. Although the analysis of nucleic acid has been successfully achieved by real-time nanomaterial fluorescence systems, there are still many shortcomings such as complex procedures or expensive instruments. To address these disadvantages, Wang’s group introduced a highly sensitive and visualized detection of nucleic acid by the combination of strand exchange amplification (SEA) and lateral flow assay strip (LFA) [131]. The system, which is possible to be widely used in areas requiring limited resource, is mainly characterized by integrating SEA with LFA (Fig. 6). There is no denying that the extremely high fluorescent signal for bioanalysis plays an irreplaceable role in these applications.
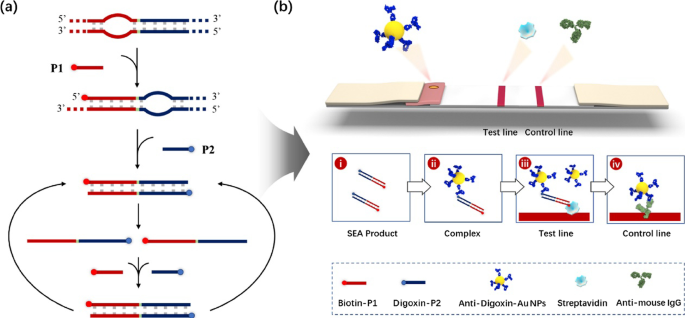
Schematic illustration of the SEA-LFA strip for the detection of nucleic acids [110]
Drug Detection
In the field of drug analysis, a facile and low-cost analytical method is always in demand for high-speed detection of specific pharmaceutical compounds. Real-time detection of drugs can be achieved with selective and sensitive fluorescent nanomaterials owing to their outstanding optical properties. In the past decade, the modification of nanomaterials has lowered their detection limit and improved their detection accuracy significantly. Recently, it is reported that ampicillin can be detected in serum sample based on aptamer, its complementary strand (CS) and gold nanoparticles (AuNPs) [132]. The limit of detection (LOD) of this method can be as low as 29.2 pM. However, there are still many limitations in the detection of drugs or target molecules in vivo. Due to low selectivity, conventional fluorescent nanomaterials inevitably generate false positive results and adverse effects in vivo. In addition, current tracking systems can hardly realize real-time tracking because of insufficient labels and excitation sources. Considering the above limitations, a new method using up/down conversion (UC/DC) PL nanomaterials has attracted increasing attention. Seo y col. reported a single-photon-driven UC/DC system which demonstrated outstanding performance in the detection of heavy metal ion (i.e. Hg 2+ ) in mussels [133]. LOD of the nanohybrids was ca. 1 nM. This system is appealing to researchers in the field of fluorescent nanomaterials for biomedical applications.
Drug Delivery
Until now, the technology of treating cancers with high efficiency and targeting function is not perfect enough. Under most circumstances, the anticancer drugs are distributed and released extensively in the body, which endangers the healthy cells and tissues irreversibly. Currently, a large variety of carriers for drug delivery have been designed. However, we can hardly supervise the distribution and result of the whole delivery process. Benefiting from the recent development of the surface modification technique, fluorescent materials capped with polymers like polyethylene glycol (PEG) can bond with drugs strongly and firmly. Then, the loaded drugs will be released in response to certain conditions such as pH, osmotic gradient and the surrounding environment. However, it should be confirmed whether the drugs are transported to the specific site or not. It’s also necessary for us to consider more details such as how much of the drugs is released in different positions. Aside from being drug carriers, fluorescent nanomaterials can also demonstrate the consequences of intracellular uptake due to their fluorescence property. QDs have been applied to monitor some important properties, such as delivery efficiency, release rate and distribution of drug molecules in vivo, which are beneficial for scientists in order to understand the specific targeting pathways of drug delivery within living cells. Duan and co-workers reported a facile pH-responsive fluorescent CDs drug delivery system [134]. Loaded with dox which is effective for gastric cancer, intracellular drug delivery and tracking could be simultaneously realized in patients (Fig. 7). The report highlighted the ability of fluorescent CDs to label and track the drug delivery process for at least 48 h, which showed a great potential in bioimaging, biolabeling and traceable drug delivery. Duan et al. designed a pH and receptor dual-responsive drug delivery system [135]. Hyaluronic acid was covalently attached to the surface of CDs, and doxorubicin was loaded by electrostatic self-assembly. In the tumor microenvironment (pH 5.6), the drug is released rapidly from the drug delivery system, while in the normal physiological environment (pH 7.4), the drug is hardly released. Endocytosis occurs when the drug delivery system reaches CD44 which is a receptor rich in tumor cells and can bind specifically to the hyaluronic acid. In addition, carbon nanotubes can be used for drug delivery by virtue of their high loading efficiency. Strong π-π interactions play a critical role in binding therapeutic agents with carbon nanotubes, which can be broke through changing external conditions, resulting in the release of drugs in specific position. Pennetta et al. functionalized single and multi-walled carbon nanotubes with a pyrrole derived compound to form a doxorubicin stacked drug delivery system. Biological studies showed that the synthesized nano-conveyors can effectively deliver the drug into cell lines and improve the therapeutic effects of doxorubicin [136].
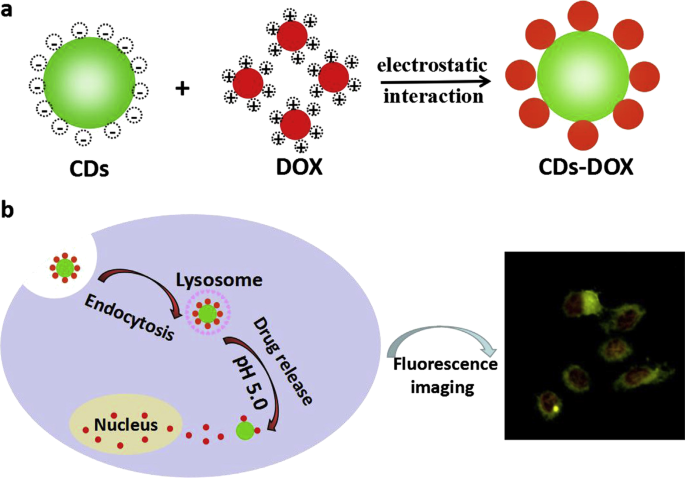
Schematic illustration of the preparation (a ) and cellular uptake (b ) of the CDs-DOX drug delivery system [113]
Photodynamic Therapy
Photodynamic therapy (PDT) is a novel therapy method for tumors which utilizes the interaction between light and photosensitizer. In PDT, reactive oxygen species (ROS) is produced from oxygen by photosensitizers in the condition of specific wavelengths of light (mostly in the area of near infrared light). The specific mechanism is presented in Fig. 8. ROS includes singlet oxygen, superoxide radicals, hydrogen peroxide, and hydroxyl radicals that possess strong cytotoxicity which cause significant destruction of tumor cells. However, there exist many defects such as limited penetration depth [137], hydrophobic properties [138], photobleaching [139], complicated procedure [140], and tumor hypoxia. PDT agents can hardly be dissolved and they will disperse extensively in vivo once they are taken, making it impossible to be targeted and selected. Fluorescent nanomaterial based photodynamic therapy developed fast in recent years [141]. Combined with the unique properties that QDs possess, such as high fluorescent efficiency and great spectral resolution, the effect of PDT can be enhanced. Barberi-Heyob, M and coworkers significantly enhanced the photodynamic efficiency with a concentration of 8 nM because of the light dose-dependent response [142]. In addition, photodynamic therapy can sometimes do harm to the skin and eyes of patients due to its photosensitive side-effect. To alleviate these adverse effects, a novel nanoparticle-based drug carrier for photodynamic therapy is reported which can provide stable aqueous dispersion of hydrophobic photosensitizers. Meanwhile, the key step of photogeneration of singlet oxygen was preserved, which is necessary for photodynamic action [143]. It is obvious that QDs combined photodynamic therapy will replace the conventional PDT someday.
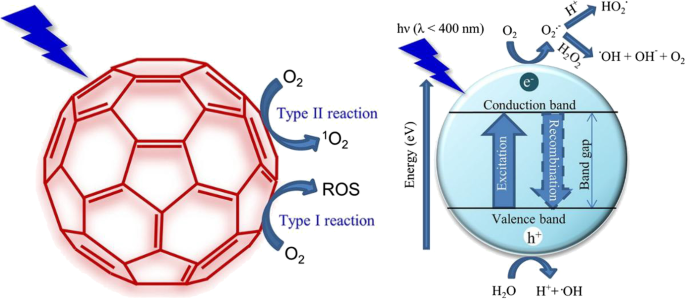
Schematic illustration of producing reactive oxygen species (ROS) for the photodynamic therapy (PDT) [119]
Challenges
Synthesis Challenges
Achieving Uniform Distribution
In the synthesis process, the diameter and size distributions of FNMs can be hardly distributed uniformly due to the agglomeration of small particles. This could be fatal to the optical properties of FNMs in biomedical application. For this reason, the applications of FNMs are still at the laboratory scale. It has been confirmed that the surface properties primarily determine the agglomeration state of the nanoparticles and their size. Therefore, surface modification is promising to achieve uniform distribution of FNMs by altering their surface properties [144]. To date, silanized QDs have been widely used because the polymerized silica coating increases the stability in buffers under physiological conditions [145]. Carbon dots synthesized by hydrothermal reaction using water-soluble base were reported to be difficult to control the size and distribution of grain boundary [146]. Khanam et al. reported a facile and novel synthetic method for the preparation of hydroxyl capped CDs using an organic base and a surfactant (Triton X-100) to modify the surface. A narrow particle size distribution at 7.2 nm was found in Raman and DLS studies, which is smaller than the majority of the particles falling within the range of below 10 nm in diameter [147].
Fluorescence Quantum Yield
Fluorescence quantum yield plays a crucial role for FNMs in their efficiency for on-demand light emission. Tunable and highly fluorescent CDs can be prepared with the surface functionalization approach. Nitrogen-doped FNMs are reported to have improved fluorescence quantum yield. With increasing nitrogen content, fluorescence quantum yield can be increased to as high as 56% at high synthesis temperature [148]. A facile strategy was also developed to tune the photoluminescent properties of CDs using a microwave irradiation, with citric acid and nitrogen-containing branched polyethyleneimine (b-PEI) as precursors. At intermediate levels of b-PEI, the CDs produced a high photoluminescence yield [149]. Lin et al. explored carbon dots with a high-fluorescence quantum yield rate synthesized from L-cysteine and citric acid by the microwave-assisted method. The obtained carbon dots exhibited a high-fluorescence quantum yield (up to 85%), which is due to the combination of amidogens and sulfydryl with carbon dots, and henceforth bringing the improved fluorescence property [150]. The above examples demonstrate that nitrogen or other electron-rich atoms like sulphur can obtain satisfying fluorescence quantum yield.
Aggregation-Caused Quenching
Fluorescent molecules can emit light with high efficiency in dilute solution. However, in concentrated solution or solid state, their fluorescence will be weakened or even disappear. This phenomenon is called Aggregation-Caused Quenching (ACQ) [151]. This problem has been puzzling scientists for almost 150 years, thus hindered the extensive application of fluorescent dyes.
In order to make effective use of fluorescent dyes, scientists have attempted many methods. Most of them focused on reducing the concentration of fluorescent dyes to prevent ACQ effect. Tang et al. discovered the phenomenon of Aggregation-Induced Emission (AIE) [152]. Based on rationally designed molecules, the fluorescence of organic molecules in solid state can be attained. Still, for more than one hundred thousand different fluorescent dyes in the world, the problem of ACQ has not been completely resolved. As long as they aggregate together, ACQ will make them lose their fluorescent properties.
It is almost impossible for high concentration or solid state FNMs to show reliable fluorescence due to fluorescence quenching. Although fluorescence quenching can be used as a sensitive signal to indicate substrate concentration in analytical chemistry, [153] in the most circumstances, however, fluorescence quenching is undesirable for FNMs because it always has considerable influence on bioimaging and biodetection. To overcome this long-standing problem, Benson et al. reported a universal solution with the discovery of a class of materials called small-molecule ionic isolation lattices (SMILES) [154]. SMILES are simple to make by mixing cationic dyes with anion-binding cyanostar macrocycles. We draw inspiration from their findings and believe that similar results can be obtained if we replace cationic dyes with cationic modified FNMs.
Application Challenges
Drawbacks of UV Light FNMs
Although FNMs realized the great-leap-forward from in vitro imaging to in vivo imaging, the emission fluorescence of most of FNMs is distributed in ultraviolet region or short wavelength visible region, which limits the optical imaging in living organisms. Moreover, use of UV light for monitoring living processes in cells and tissues has some potential drawbacks as long‐term irradiation of living cells may cause DNA damage and cell death. Therefore, the development of FNMs in near-infrared region is urgently needed in the future. Although NIR FNMs have deep tissue penetration, NIR detectors and filters are needed as the excitation and emission wavelengths are too close to each other, which restricts their range of application.
Interference in Biological Environment
Almost all biological tissues will produce significant autofluorescence under short wavelength, UV and visible light radiation [155]. Autofluorescence reduces the signal‐to‐background ratio and often interferes seriously with the visual effects. Some substances in the substrate of biological tissues also have great influence on the fluorescence, which reduces the selectivity of FNMs significantly. Until now, although the application of FNMs in mice showed acceptable outcomes, it is still difficult to achieve similar results in larger mammals. Much higher luminous efficiency under low power density excitation is required to avoid the background signal interference. Furthermore, temperature and pH conditions of the biological environments strongly affect the fluorescence of some substances as well. Therefore, satisfying fluorescence of FNMs at 37 °C and physiological pH should be guaranteed. It's worth noting that the pH in tumor is lower than normal tissues. Hence, fluorescence with high selectivity in acid environment will improve the efficiency of FNMs.
Biocompatibility
Biocompatibility refers to materials or systems that are nontoxic, safe and not causing physiological or immunological reactions. QDs with unique quantum confinement effect and electro-optical properties are attractive for biomedical applications. However, toxic effects of traditional semiconductor QDs made of heavy metal ions have serious safety concerns for their undesired environmental or health effects. In the purpose of circumventing this problem, core–shell structure modification of QDs by using biocompatible ligands or polymers is one way to effectively minimize toxic effects of traditional QDs. Furthermore, scientists are searching for heavy metal-free QDs formulations. Non-toxic or less toxic carbon dots and silica nanoparticles have shown their potential as the ideal FNMs for biomedical applications. Impurities brought in the process of syntheses may influence the biocompatibility of fluorescent nanomaterials. In order to reduce the influence of toxic impurities, green synthesis methods have been arousing the interest in biomedical fields. Chowdhury et al. utilized cacao extract which is a natural product as a reducing and stabilizing agent in the synthesis of gold nanoparticles [156]. Oxalic acid, as a constituent of cacao, can reduce Au 3+ in HAuCl4 to metallic gold and stabilize the resultant nanoparticle colloidal solution. In vitro studies suggested that the cacao derived gold nanoparticles are biocompatible and suitable for biomedical applications. For MOFs, appropriate metal ions and ligands must be selected to lower the toxicity. Wang et al. employed Fe, Ti and Zr as constituents of MOFs, which are harmless and even beneficial elements to the body [157]. In vitro studies indicate that the proposed material has good biocompatibility and safety in biomedical application. What’s more, it is necessary to consider whether the difference in composition, surface charge, or modified group will have different biological effects. Taking these factors into account, we can improve the biocompatibility of FNMs with rational design.
Conclusions
Benefiting from the unique properties of fluorescent nanomaterials, some limitations and barriers of conventional materials and methods in biomedical applications can be broken through. In this review, we comprehensively present the synthesis methods and applications of fluorescent nanomaterials. The advanced synthesis methods can offer us the fluorescent nanomaterials with ideal morphology, size ranges and structures. Meanwhile, the more convenient syntheses can lower the manufacturing cost of fluorescent nanomaterials, which is critical to their widespread applications in biomedical fields. Based on the improved synthesis techniques, the performance of fluorescent nanomaterials is bound to leap in their applications. With the development of fluorescent nanomaterials, bioimaging, biodection, drug delivery and photodynamic therapy will be more widely applied in the diagnosis and treatment of diseases. Finally, challenges in synthesis and biomedical applications point out exiting questions and developing direction. We hope that this review can bring some new insights to the development of fluorescent nanomaterials.
Availability of data and materials
All data and materials are available without restrictions.
Abbreviations
- FNMs:
-
Fluorescent nanomaterials
- PL:
-
Photoluminescence
- CDs:
-
Carbon dots
- QDs:
-
Quantum dots
- b-PEI:
-
Branched polyethyleneimine
- SWCNTs:
-
Single-walled carbon nanotubes
- CQDs:
-
Carbon quantum dots
- GQDs:
-
Graphene quantum dots
- NCQDs:
-
Nitrogen-doped carbon quantum dots
- NSCDs:
-
Nitrogen and sulfur doped carbon dots
- QY:
-
Quantum yield
- MCDs:
-
Multi-doped carbon dots
- MWCHTs:
-
Multi-walled carbon nanotubes
- GO:
-
Graphene oxide
- rGO:
-
Reduced graphene oxide
- CVD:
-
Chemical vapor deposition
- AuNPs:
-
Gold nanoparticles
- Ag NCs:
-
Ag nanoclusters
- Cu NCs:
-
Cu nanoclusters
- NPs:
-
Nanoparticles
- TEOS:
-
Tetraethylorthosilicate
- MR:
-
Magnetic resonance
- COFs:
-
Covalent-organic frameworks
- TNP:
-
2,4,6-Trinitrophenol
- MOFs:
-
Metal organic frameworks
- ACQ:
-
Aggregation-caused quenching
- NIR:
-
Near infrared
- SEA:
-
Strand exchange amplification
- LFA:
-
Lateral flow assay strip
- CS:
-
Complementary strand
- LOD:
-
Limit of detection
- UC:
-
Upconversion
- DC:
-
Downconversion
- PDT:
-
Photodynamic therapy
- ROS:
-
Reactive oxygen species
- AIE:
-
Aggregation-induced emission
- SMILES:
-
Small-molecule ionic isolation lattices
Nanomateriales
- Sensores y procesadores convergen para aplicaciones industriales
- Qué sigue para la impresión 3D:aplicaciones biomédicas
- Los cinco problemas y desafíos principales para 5G
- Nanopartículas para la terapia del cáncer:avances y desafíos actuales
- Compuestos de grafeno y polímeros para aplicaciones de supercapacitores:una revisión
- Hacia los nanofluidos de TiO2:Parte 2:Aplicaciones y desafíos
- Últimos avances y aplicaciones en la tecnología de IoT
- EconCore otorga licencias a Fynotej para aplicaciones automotrices de NA y avances en termoplásticos de alto rendimiento (HPT)
- Accionamientos para refrigeración industrial y aplicaciones de refrigeración industrial
- Tornos giratorios para aplicaciones de taladrado y torneado
- Comprensión de los beneficios y desafíos de la fabricación híbrida